The Sun and the Sunspots
Structure of the Sun
The Sun is a gaseous body composed mainly of hydrogen and helium. We can recognize an internal nucleus with a radius of approximately 150,000 km within which the density of matter and the temperature (approximately 15 million degrees) are so high as to allow the triggering of nuclear fusion reactions which produce the enormous energy that the sun shines.
Around the nucleus we have the radioactive zone (so called because the energy emitted by the nucleus propagates through radiation) surrounded by a convective zone in which the energy propagates through convective cells, that is, through hot currents that rise towards the surface and cold currents that descend towards the center.
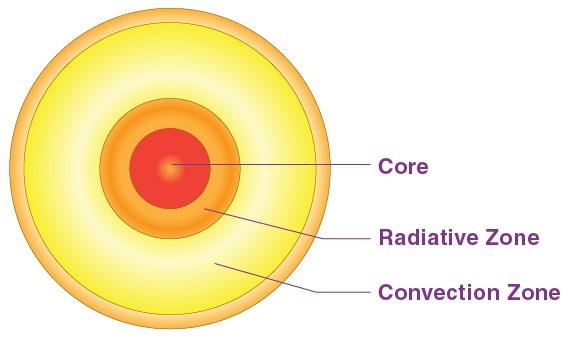
We have thus reached a distance of approximately 700,000 km from the center of our star, reaching the photosphere. The Sun is a gaseous body, so it would be improper to speak of a surface, but the photosphere is defined as such, given that it is the innermost area that we can see, since it is here that part of the energy is transformed into photons which make the underlying layers are invisible.
The Sun also has an "atmosphere" made up of the chromosphere and the corona. The latter extends up to 100 solar radii, equal to half the distance from the Earth to the Sun. Chromosphere and Corona are not normally visible as they are far less luminous than the photosphere, but they can be clearly observed during total eclipses, when the moon masks the brightness of the photosphere.
Hundreds of pages could be filled describing the structures of the Sun, but here we will focus on phenomena related to the photosphere as these are easily observable with a small instrument.
The photosphere
The photosphere does not appear uniform. The Sun appears brighter at the center than at the edge. The darkening of the edge, particularly evident in photography, is due to the fact that in this region the highest and coldest layers of the photosphere are observed through perspective.
On the solar disk you can then observe darker, rounded areas: the sunspots. The spots were observed even before the invention of the telescope, being the only structure of the photosphere which in particular conditions (sunset or slightly veiled) is visible even to the naked eye. The real nature of this structure remained unknown for a long time, so much so that until 1610, when Galileo was able to observe them by adopting the projection technique, it was believed they were due to effects of the earth's atmosphere or optical illusions. Not even after the telescopic observation did they want to admit the correlation between spots and the Sun: in order not to violate the Aristotelian prejudice of an immutable and incorruptible celestial world, the phenomenon of spots was attributed to the transit of hypothetical planets within the orbit of Mercury on the solar disk.
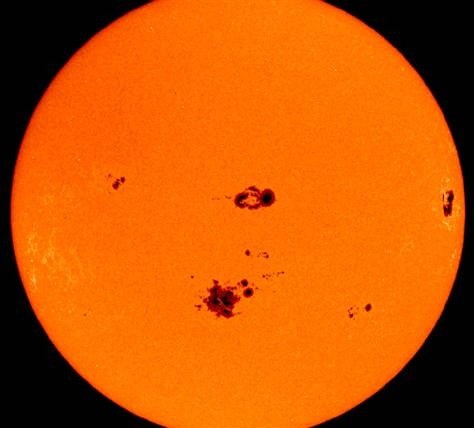
In reality, sunspots are colder areas than the rest of the photosphere (4600 degrees compared to 6000 in the photosphere); this difference in temperature means that by contrast the spots appear dark to us, but if we could observe them individually they would also appear bright. The drop in temperature recorded near these regions is due to very intense magnetic fields which disturb the energetic particles emitted by the Sun.
The spots are composed of two parts: the shadow, darker, placed at the center of the structure and surrounded by a ray called penumbra of a lighter colour. Shadow and penumbra cover a surface with a diameter between 7,000 and 50,000 km. Usually the spots are not isolated, but gathered in more or less numerous groups.
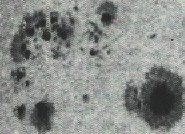
If we extend our observation to several days we can observe that they move along the solar disk, revealing the rotation of our star. To carry out this type of observation it is necessary to define reference points on the solar disk; these are obtained by letting the image of the Sun flow through the eyepiece: the direction followed by the spots will determine in a first approximation the East – West connection and consequently the perpendicular to it passing through the center will allow us to identify the North and South poles. Performed this operation we will be able to observe that the spots close to the equator complete a rotation in a shorter time than those of higher latitude; this fact demonstrates the differentiated rotation of the star which is typical of a gaseous body.
In addition to moving on the solar disk, the spots change shape and size day by day following a life cycle that takes them from birth to extinction. The cycle begins with the formation of a very small spot without penumbra called a pore.
The pore evolves rapidly, enlarging and developing the penumbra. After a few days, a little further east, other small spots develop; This results in the formation of a group made up of p-type spots (the first born) and f-type spots (the last born). The group grows in size and develops new spots located between the p and the f which in the meantime tend to move away. The group reaches its maximum extension around the tenth day when the period of decay begins characterized by the reduction and disappearance of the central spots and the rapprochement of the p and f spots, until only a small spot without penumbra remains (the f type spot) which will be reabsorbed by the photosphere.
The one just described is an ideal life cycle that is not always completed: many stains do not go beyond the pore stage, others appear isolated, still others vanish after a few days; their life is therefore variable and can range from a few hours to a few months.
If we observe the solar disk for several days we realize that the spots are variable not only in shape and size, but also in number; we will therefore have days in which numerous groups can be clearly seen and others in which the photosphere will appear completely clear. As we have seen, the spots are caused by the magnetic activity of our star, so it may be interesting to consider the number of spots as an index of the Sun's activity. An index of this type was introduced in 1848 by R. Wolf and since then it has became the standard for measuring solar activity. The relative Wolf number is given by the formula R=k(10g+f), where f indicates the number of single spots, g the number of groups and k is a factor that depends on the instrument used and on the atmospheric and observer conditions; to obtain a reliable value of k, simply compare the values obtained by us with the official ones for a few days. Analyzing the values of this index over the long term, a cyclical trend of approximately 11 years can be observed.
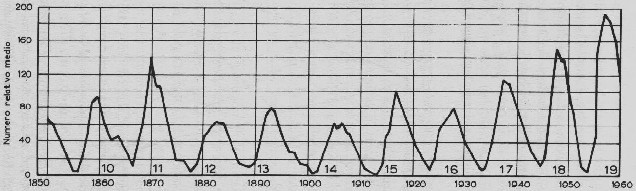
The eleven-year cycle not only influences the number of spots, but also their position with respect to the equator: at the beginning of the cycle the spots will concentrate at a latitude close to 40 degrees and drop to 5 degrees in the maximum period (the spots do not never occur outside this range).
By plotting the latitude versus time you will thus obtain a diagram with a particular shape called a butterfly diagram.
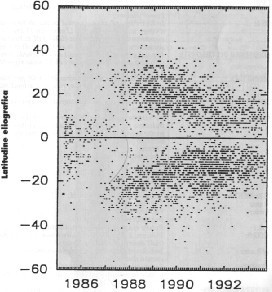
Photospheric faculae are regions of the photosphere that are hotter than the rest of the surface, so they appear lighter to us; they are more easily observable near the edge of the solar disk due to the darkening which increases the contrast.
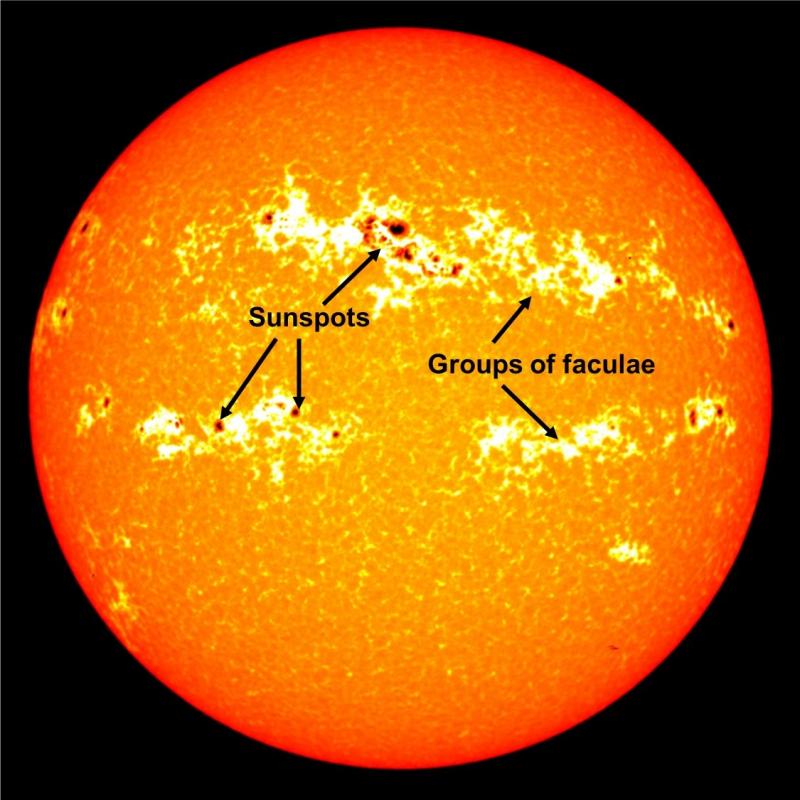
The faculae have several characteristics in common with the spots: they too are associated with magnetic fields (of lesser magnitude which allow an increase in the efficiency of the photosphere), they follow the eleven-year cycle and move towards the equatorial regions in periods of maximum; they will therefore occupy the same areas of the photosphere occupied by the spots.
Unlike sunspots, photospheric faculae have a larger extension (10 billion square kilometers) and a lifespan three times longer; they appear approximately three days before the formation of the spot (they can therefore be used as an indication of the formation of a new group) and evolve following the movements of the spots, becoming increasingly longer in longitude and distorting due to the differential rotation of the star for then dissolve very slowly. There are particular types of faculae that are found beyond the boundary of 40 degrees of latitude, the so-called polar photospheric faculae; these, obviously not associated with spots, usually do not exceed three thousand kilometers in diameter and have a lifespan of between a few minutes and a few days. Unlike the other faculae, the polar faculae behave in the opposite way to the solar cycle, with maximum frequency and intensity in the minimum periods and minimum in the maximum periods.
In particularly favorable seeing conditions it is possible to see the photospheric granulation.
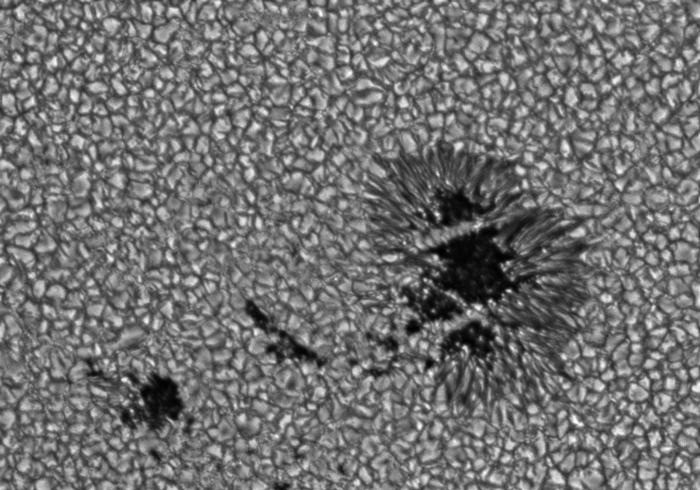
It is characterized by irregular polygons with a diameter of only 1000 kilometers interspersed with darker areas which are believed to represent the emergence of convective cells at the photosphere level: the lighter grains represent the hottest matter reaching the surface, while the lighter areas dark would indicate the regions in which the cooled matter sinks back towards the center of the star. The life of each grain is very short, on the order of a few minutes.
The observation of the granulation is made very difficult due to the atmospheric turbulence which does not allow us to see such minute details, therefore, on a professional level it is carried out using instruments placed on satellites or sounding balloons. Granulation was observed for the first time in 1748 by James Short who baptized it with the name still used today of "grains of rice".
The rarest and most impressive phenomenon we can witness when observing the photosphere is the flare. Flares consist of a temporary and localized heating of the photosphere which can release energy equal to one tenth of the total energy emitted by the Sun. As we have said, this is a very rare event to observe at a photospheric level, given that it is a phenomenon usually associated with the solar atmosphere (chromosphere and corona), so only the most intense flares will also be able to involve the photosphere. A flare has a life of a few minutes, but in this short period of time it manages to emit a very high number of energetic particles which, once they reach the Earth, interacting with the atmosphere, can cause intense magnetic storms which manifest themselves with auroras at low latitude, disturbances in radio communications and damage to satellites.