The importance of the water for the origin of life on Earth
About 4.5 billion years ago, the cosmic gases that made up the Earth solidified: the first rocks were formed and the intense volcanic activity produced an atmosphere rich in water vapor (H2O), carbon monoxide and anhydride carbon dioxide (CO and CO2), nitrogen (N2) and hydrogen cyanide (HCN).
The planet's surface was so hot that the rain evaporated as soon as it hit the ground.
When the planet cooled about 3.5 billion ago, water went from a gaseous to a liquid state, allowing oceans to form.
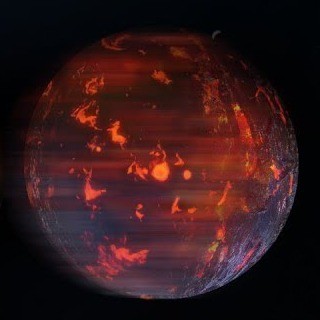
A hypothesis on the origin of life was formulated by the Russian biochemist AI Oparin in 1932 who hypothesized that the organic molecules that are the basis of the constitution of living beings, had originated in an environment with little free oxygen and when the primordial atmosphere was consisting of water, hydrogen, ammonia and methane (the compounds containing the 4 chemical elements C, H, O, H which make up about 97-99% of the living substance).
The energy needed to obtain the organic molecules by splitting the gaseous material came from the heat of the Earth itself, from the ultraviolet rays of the Sun and from the electrical discharges produced by the various hurricanes.
This hypothesis was tested in the laboratory by the American Stanley L. Miller in 1953 , who simulated the conditions of the primitive Earth in a sterile flask that contained water and gases believed to be present in the atmosphere. By passing electrical discharges, similar to those of lightning, into this environment, he observed the formation of various types of carbon compounds, including molecules of the simplest amino acids, the building blocks of proteins, the most important constituents of living beings.
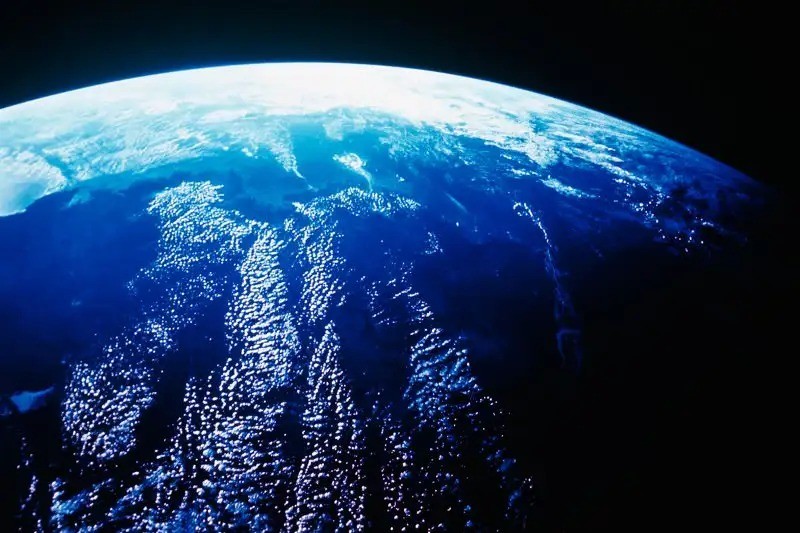
The organic compounds formed in the atmosphere were captured by the rain and ended up in the oceans; these became increasingly rich mixtures of organic molecules so as to compare them to a hot broth.
For life to begin, these simple organic compounds needed to associate into more complex structures.
Some scientists believe that the small organic molecules contained in the “primitive broth”, in contact with clayey surfaces, could have spontaneously organized themselves into ordered structures of large dimensions.
The organic molecules, agitated by the waves and currents, would have given rise to particular aggregates known as coacervates, which had some similarity with the current cells (they were surrounded by a two-layer membrane and tended to incorporate organic substances from the environment, therefore could grow).
The coacervates, despite their complexity, were not yet living beings, which appeared on Earth only the day in which a coacervate, having reached the maximum dimensions compatible with its existence, instead of splitting into two smaller parts, divided into two perfectly identical parts, that is, it self-doubled.
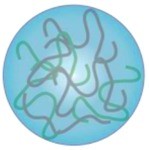
The first living cells were anaerobic (oxygen had not yet formed), heterotrophic and dependent on organic compounds present in the environment as a source of energy. They were probably single-celled and prokaryotic like many bacteria today (fossils of these microscopic organisms have been discovered in rocks dating back 3.5 billion years).
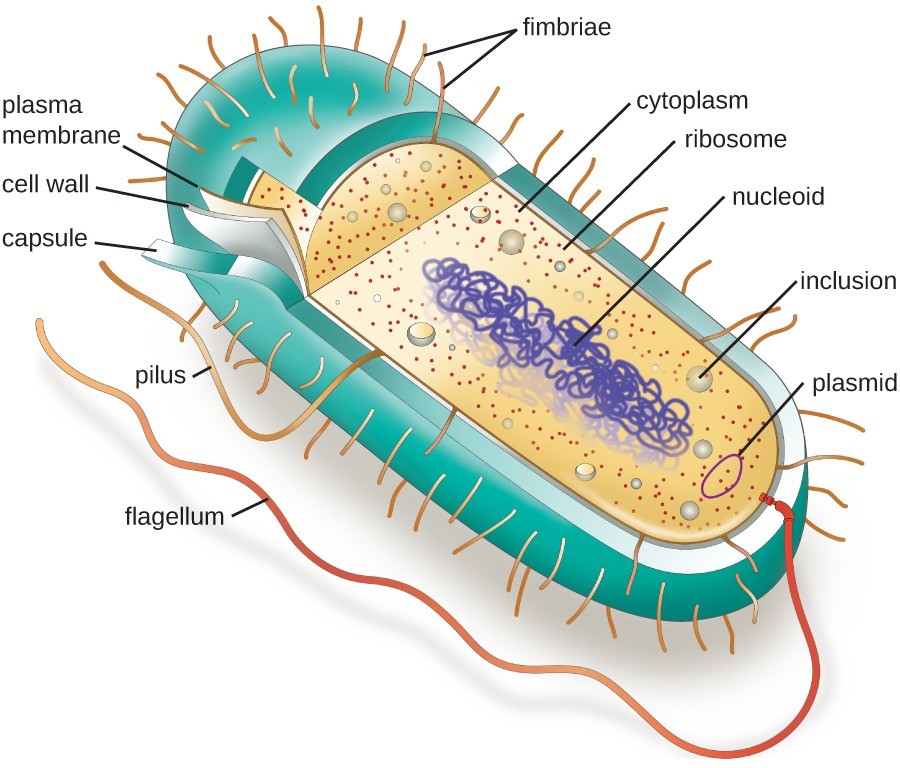
Over time, however, preformed organic substances began to decline due to two factors
- the continuous absorption by these primitive heterotrophs
- because their rate of formation became lower: it is possible that the ultraviolet radiations coming from the Sun have split the water so that small quantities of free oxygen have collected in the upper layers of the atmosphere. Oxygen (O2) turned into ozone (O3) which began to act as a filter for ultraviolet radiation.
Selective pressure for the search of new energy sources may have eventually led to the development of primitive forms of photosynthesis.
The first photosynthetic organisms used hydrogen sulphide (H2S) instead of water, releasing sulfur instead of oxygen and spread rapidly giving rise (about 3.5 billion years ago) to sulfur deposits called Stromatolites.
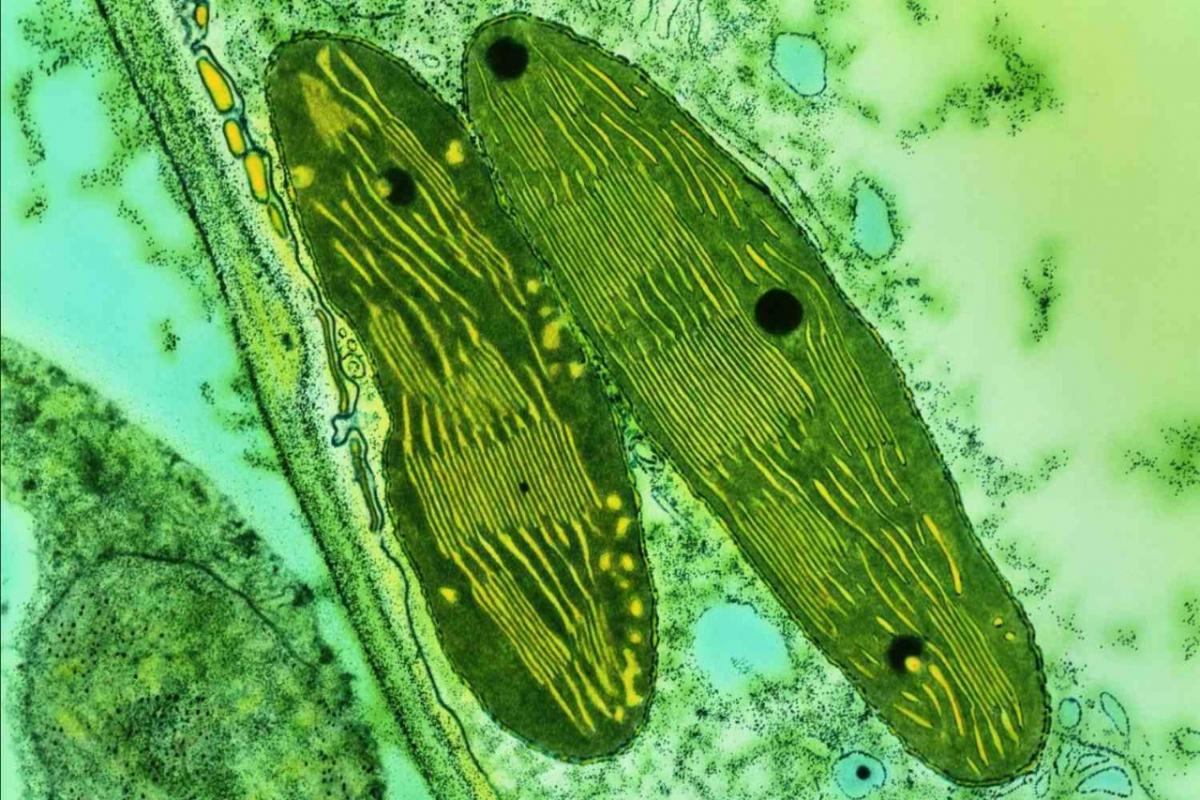
About 3 billion years ago, a new group of prokaryotes appeared and began to photosynthesize like plants today, releasing oxygen as a by-product.
Water and its biological role
Life began in water, and it cannot exist without water. Just as water is critical to life on a global scale, so is it on a cellular and molecular level.
Water constitutes about 70-90% of all living cells.
All reactions in life are influenced in many important ways by the chemistry of water. The water content of an organism is related to its age and its metabolic activity (it is at its maximum in the embryo and progressively decreases in the adult).
As for its origin, water must be distinguished in organisms in exogenous water, coming from the outside with food (in aquatic organisms also through the surface of the body), and in endogenous water which is formed during metabolic reactions.
Its biological importance is linked to its many properties:
- It is an excellent solvent of gases (such as O2 and CO2), of mineral salts and organic substances, and is the dispersing phase of macromolecules (proteins and lipids)
- It represents for most cells the means of transport by virtue of which the various nutrients can be absorbed from the outside and waste products can be expelled.
- The chemical reactions that take place in the cells are essentially carried out in an aqueous environment.
- It directly takes part in important chemical reactions such as those of hydrolysis and synthesis by dehydration
- Together with mineral salts, it contributes to keeping the cells turgid.
Its properties are due to the structure of its molecule which is made up of two hydrogen atoms joined to an oxygen atom by simple covalent bonds; It is V-shaped and highly polar. Hydrogen atoms carry partial positive charges, and the oxygen atom represents a region of partial negative charge.
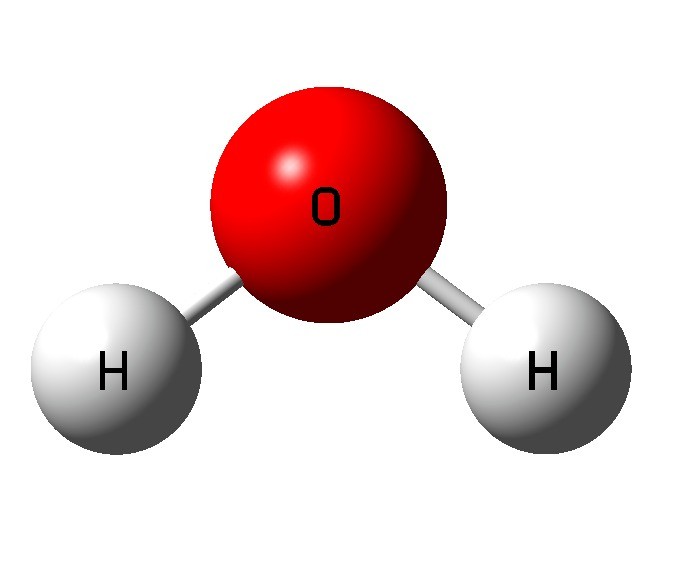
It is a polar molecule, having one region with a positive charge and one with a negative charge. This is precisely because the hydrogen atom is covalently linked with an atom with a high electron affinity so that the shared electron pair is more strongly attracted to the oxygen atom than to the hydrogen one.
The whole molecule is electrically neutral, but the electrons are not evenly distributed in the molecular orbitals. They are strongly attracted to the large positively charged oxygen nucleus and therefore the electron cloud is denser in the region of the oxygen core than near the hydrogen nuclei.
The water molecule as a dipole
The molecule can be represented with a positive pole corresponding to the hydrogens and a negative pole corresponding to the oxygen atom. The local charges compensate each other so that the result is a molecule which on the whole is electrically neutral; but the water molecule actually behaves like a miniature dipole.
Hydrogen bond
Between two water molecules, due to this separation of charges, a force of attraction occurs between the positive pole of one and the negative pole of the other. This electrostatic attraction is called hydrogen bond (it is formed every time that a hydrogen atom, already covalently bonded to an atom - more electronegative - of nitrogen or oxygen, binds to another nitrogen or oxygen atom, located in the nearby space, but belonging to another molecule).
The stabilization of hydrogen bonds between different molecules forces them to assume a precise spatial arrangement. For this reason these bonds are defined as directional bonds.
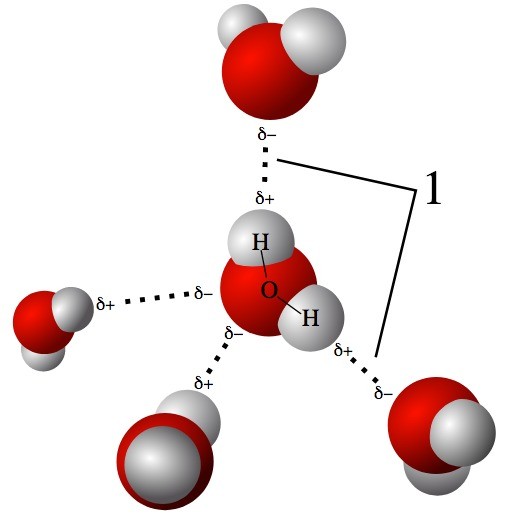
Since the electrons around the oxygen atom are thought to be arranged like the vertices of a tetrahedron, each water molecule can theoretically form hydrogen bonds with 4 surrounding molecules.
In liquid water at room temperature, each molecule is believed to be hydrogen bonded to 4 other water molecules. The hydrogen bonds are rather weak but, due to their large number, they give liquid water a great internal cohesion, even if as a consequence of the continuous rupture and reconstruction of the hydrogen bonds, water in the liquid state is not viscous but very fluid.
Each hydrogen bond has an extremely short duration. A possible increase in temperature breaks a greater number of hydrogen bonds at each instant and the free molecules move away to the surface carrying heat. The cold instead immobilizes each molecule in space, blocking it through the hydrogen bonds, to 4 other molecules to form the regular crystal lattice structure of the ice.
Water as a solvent
The polarity of water makes it an excellent solvent for a large variety of (hydrophilic) substances.
Any substance that has a polar nature (an asymmetrical distribution of charges) tends to interact with the water molecules with the same type of interactions between charges, responsible for the reciprocal relationships between the water molecules. These polar substances are called hydrophilic (water-loving). If molecules or ions of a hydrophilic substance interact with water more strongly than with each other, that substance dissolves in the water.
Water is an excellent solvent and this property derives from the interactions that are established between the water molecules and the particles (molecules or ions) dissolved in it.
An example can be given by dropping a crystal of table salt (Sodium Chloride) into the water. In the salt crystal, the Na and Cl ions with opposite electrical charges alternate regularly. This is a relatively stable situation, where each ion inside the crystal is surrounded by 6 ions of opposite charge. However, the ions on the crystal surface are surrounded by a few opposite charge partners and are partially exposed. When the crystal is poured into water the ends of the negatively charged molecules are attracted to the exposed sodium ions, while the positively charged ends are attracted to the exposed chloride ions.
Since sodium and chloride ions interact more strongly with water than with neighboring ions in the crystal, they tend to leave the surface of the crystal and surround themselves with many layers of oriented water molecules, called hydration spheres. This exposes a new layer of ions in the crystal and the process continues until the crystal has melted.
The sodium and chlorine ions surround each other with a halo of water molecules oriented differently according to the charge of the ion, so they are spaced apart.
With this mechanism, water brings into solution not only small ions, but also more or less complex molecules.
A sugar crystal dissolves quite similarly when poured into water. But the interactions that stabilize a sugar crystal and cause it to dissolve in water are quite different from those involving the salt crystal. Sugars do not contain ionic groups, but possess numerous polar - COH groupings in which the highly electronegative oxygen atom draws the electron doublets on itself away from carbon and hydrogen. These polar groups form hydrogen bonds with each other stabilizing the sugar crystal. They form even stronger hydrogen bonds with water; so when a sugar crystal is poured into water, it dissolves because the hydrogen bonds between the sugar molecules are broken and replaced with hydrogen bonds between sugar and water.
Most of the substances of life are hydrophilic, they possess both ionic groups and polar regions capable of forming hydrogen bridges with water. Water is a powerful solvent for these substances, it dissolves them, brings them closer, allowing them to react with each other in the chemistry of life .
Consequences of the hydrogen bond
1) Water is highly cohesive
According to its chemical formula, water is a small molecule, but due to the large number of hydrogen bonds that hold the molecules together, it has many of the properties of a much larger molecule. The tendency of molecules to stick together is called cohesion and water is one of the most cohesive fluid. Consequence of cohesion is its high surface tension (an object that penetrates a mass of water must break the hydrogen bonds between adjacent molecules and the surface of the water shows considerable resistance to such penetration).
2) Water has a high heat capacity
When any substance absorbs heat, the kinetic energy of its molecules increases and its temperature rises. Water has a higher thermal capacity than any common liquid, that is, it can absorb a huge amount of heat by slightly increasing its temperature.
This is because much of the absorbed heat is spent not on setting molecules in motion, but on exchanging hydrogen atoms between adjacent molecules. The oxygen atoms of neighboring molecules change mates: the H atom covalently bonded to an oxygen atom and with hydrogen bond to a second oxygen atom exchanges them to bond with hydrogen bond to the first and with covalent bond to the second.
The energy absorbed in the form of heat is dissipated in small movements of hydrogen atoms rather than in larger movements of entire molecules, so the temperature rises less than one would expect.
An important biological consequence of the high heat capacity of water is that cells do not overheat until die when carrying out the reactions of life (most cell reactions occur with the production of heat).
3) Water has a high heat of evaporation
To transform from liquid to vapor, the molecules of water must be removed from the surface layer and the hydrogen bridges that hold them together must be broken. Due to the large number of them and their strength, water has the highest heat of evaporation of any other substance that is liquid in nature at room temperature.
An important biological consequence is the large cooling effect of a modest evaporation. Each molecule of water that passes from the liquid to the gaseous state carries with it all the thermal energy necessary to break the hydrogen bonds with the neighbouring molecules. The human body loses heat by evaporation through breathing and perspiration.
4) Ice floats on water
Most substances become denser as their temperature drops. As the kinetic energy decreases and the molecules move more slowly, they tend to tighten by forces of attraction.
This is also true for water up to a temperature of 4 ºC, at which it has the highest density. As the water freezes, its density drops sharply.
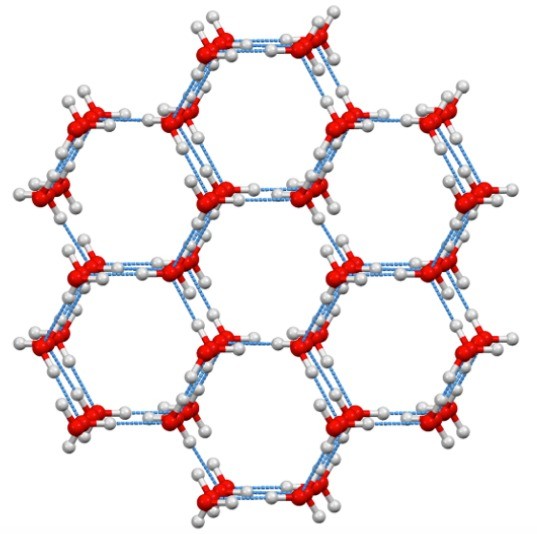
This behavior is due to the ice crystal architecture: to achieve a regular structure in which each molecule is linked to 4 others, a crystal lattice must be built containing large empty spaces. In liquid water, with its less regular structure, these spaces do not exist. But as the water freezes and each molecule is pushed into place by the hydrogen bonds, gaps form and the density decreases, so the ice is less dense than water and floats.
The biological significance of this is that when a lake's water begins to freeze, ice forms on the surface. Since it conducts little heat, it tends to isolate the water below to prevent the lake from freezing completely in order to allow the survival of the species contained in it.
As for the interaction of water with other substances, in addition to its ability to be an excellent solvent, we must also consider its capillary action.
Capillary action
The molecules of some substances, although hydrophilic and capable of interacting with water, interact even more strongly with each other and show no tendency to dissolve. One such substance is cellulose which is a large molecule made up of sugars arranged in such a way that adjacent cellulose molecules form many hydrogen bonds between them.
Cellulose fibers show no tendency to dissolve in water, however polar groups on their surface form hydrogen bonds with it. For this reason, paper towels absorb water without melting.
The formation of hydrogen bonds is also responsible for capillary action: a process that causes pipes made of hydrophilic (polar) substances such as cellulose to rise.
Capillary action involves both the cohesion of water and the attraction of water to hydrophilic substances and cannot occur in pipes made of non-polar materials. When a hydrophilic tube is immersed in water, its molecules bind to its walls by means of hydrogen bonds or by charge-charge interaction. Since the water molecules move with a certain kinetic energy, it happens that gaps in them that are found on the surface, come into contact with the highest region of the tube, attach themselves to it and pull up other molecules behind them (which of course are linked to them through the hydrogen bonds of water).
In very narrow tubes of highly hydrophilic material (such as xylem, the water-carrying channels in plants, which are lined with cellulose), water can be brought to considerable heights by capillary action alone.
Water as a reagent
So far we have dealt with the physical properties of water and its interactions with other molecules as a solvent. But water is an important protagonist in the chemical reactions of life: many processes use water as a raw material or produce water as a final product:
- Synthesis by dehydration: the formation of biological molecules through the polymerization of monomers is always accompanied by the formation of water. During protein synthesis, the peptide bond between two amino acids that occurs between an amino group and a carboxylic group involves the release (dehydration) of a water molecule.
- Hydrolysis: when we eat an apple, we cannot incorporate its macromolecules directly into our cells, but we can reuse the structural units that those macromolecules were made of to build our own macromolecules.
In order to be reused, the polymers are broken into their constituent parts by a process that is the inverse of the synthesis by dehydration: the elements of water are added to the monomers, freeing them to be then used. Two monomers can be separated by adding a hydrogen atom to one of them and an OH group to its neighbor.
This process is called hydrolysis (splitting by water).
Hydrolysis is the most important mechanism that occurs in the digestive tract after a meal has been eaten.
Most organisms, both autotrophic and heterotrophic, are capable of storing food reserves, when these are abundant, by synthesis by dehydration. These reserves are then mobilized for use when needed thanks to hydrolysis. Hydrolysis is also used to break down the aged cells of an organism so that the monomers that make them up can be reused by new growing cells.